OPTICAL
PROPERITES OF GEMS
Optical
properties are those which are related to the behavior of light,
on, or in, a gemstone.
Some
of these can be seen, and even quantified, with the naked eye alone.
Three such characteristics are: luster, transparency, and color.
The study of these factors, and their use in gem identification
and evaluation, is sometimes called optical gemology.
Other characteristics are revealed, or measured, only through the
use of special instruments. Some of these include: refractive index,
optical character, birefringence, pleochroism, dispersion, reaction
to ultraviolet light and selective absorption. When these properties
of gems are analyzed and measured, one is engaging in laboratory
gemology.
Luster :
The
luster of a gemstone is comprised of the quantity and quality of
the light reflected from its surface. There is an inherent, potential
luster possible for each species and variety of gemstone. The actual
luster, on any individual piece, however; may be less than this,
due to the skill level of the lapidary or facetor, the presence
of inclusions, or various chemical or physical changes, such as
oxidation or abrasion, that can affect the surface.
The
names, which have been given to the various lusters seen in gems,
are derived from their resemblance to familiar surfaces. (The prefix
sub- indicates "just less than".) Some lusters are so
embodied by a particular stone, that its appearance is named for
that stone, as in the case of adamantine luster (adamas = Greek
for diamond), and pearly luster. Looking through either of your
textbooks at the descriptions of the various gems will convince
you that a substantial majority of gems have a glass-like or "vitreous"
luster.
Look
at the picture of the fire agates below and compare what you see
on their surfaces to that which you'd see on a freshly washed and
dried drinking glass keeping that image in mind should help greatly
in estimating the luster of a gem.
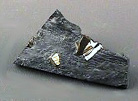
Pyrite
(in shist) : Metallic
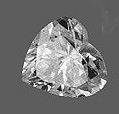
Diamond
: Adamantine (like diamond)
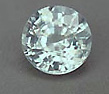
Zircon
: Subadamantine
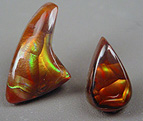
Fire
Agate: Vitreous (like glass)
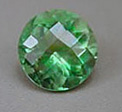
Fluorite
: Subvitreous
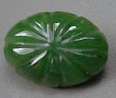
Nephrite
Jade : Greasy
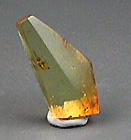
Amber
: Resinous
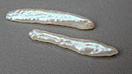
Pearl
: Pearly
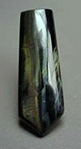
Tiger's
Eye : Silky
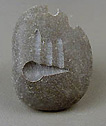
Granite
: Dull
Transparency
:
Technically
known as "diaphaneity", the degree of transparency of
a gemstone is one of its most directly observable and familiar characteristics.
Transparency
(or lack of it) is dependent on how much light gets through the
gem, and is affected not only by the chemical and crystalline nature
of the gem, but also by its thickness and, as in the case of luster,
by inclusions, and its surface condition. In the discussion and
examples that follow below, we will be looking at the "potential"
maximum transparency of a species in general, rather than the actual
transparency of any individual specimen.
When
light hits the surface of a gem, there are only three fates for
it (with respect to transparency). Various portions of the total
amount of light will be reflected, absorbed or transmitted. The
proportion in each category will determine the transparency of that
gem.
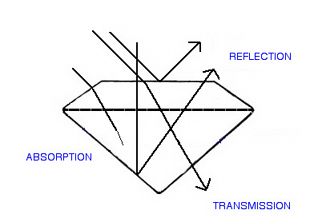
Three
fates for light hitting a gem : It can reflect (be returned) from
the surface or interior of the gem, it can be absorbed by the gem,
or it can be transmitted through the gem
Reflection :
Light
is reflected when it hits an exterior or interior surface of the
gem and is bounced back off, or out of, the gem, in the direction
of the observer.
Absorption :
When
light enters a gem and does not exit, we say it has been absorbed.
Light is a form of energy, and energy does not just disappear, instead
the visible light has been converted to a non-visible form of energy,
in most cases, heat.
Transmission :
Light
that travels through the gem and exits in a direction other than
that of the observer, is said to have been transmitted.
The issue of transparency (with the factors of reflection, absorption
and transmission) is actually more complex than it may seem at first,
because it is intimately linked with the color characteristics of
a gem. For the time being, however; we can be satisfied with the
following descriptions:
Opaque :
No
light is transmitted.
Translucent :
Some
light is transmitted.
Transparent :
A
high proportion of the light is transmitted.
The term "semi" is sometimes added to describe intermediates,
and gives additional categories beyond the basic three.
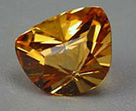
Citrine
: Transparent
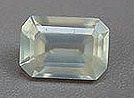
Prehnite
: Semi-transparent
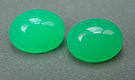
Chrysoprase
: Translucent
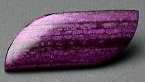
Sugilite
: Opaque
Within
any particular species of gem, it is often the most transparent
pieces which are the most valuable. For example, in chrysoprase,
shown above, which is generally semi- to fully translucent, one
finds occasional pieces that are semi-transparent. These are greatly
admired and sell for higher prices. The same can be said of nephrite
and jadeite jades where price (within the same color) can escalate
dramatically based on nuances of transparency. Likewise, in gems
that are usually opaque, like the sugilite pictured above, the
occasional semi-translucent to translucent piece (called "gel
sugilite"), is highly prized.
Color :
The color of a gem is determined by selective absorption
of some of the wavelengths of light. We know that what appears
to us as white (or colorless) light is actually made up of light
of various colors. Issac Newton was the first to demonstrate this
back in the 17th century.
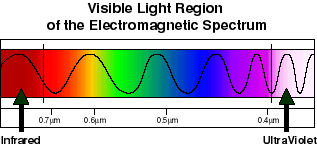
Scientists
in later years, were able to show that the color of light is a function
of its wavelength. In the diagram above, a wave-form representation
shows the relative distance from crest to crest (wavelength) of
the various components of white light. Notice that these distances
increase toward the red end of the spectrum and decrease toward
the violet end. The wavelengths are very small, and we do not have
everyday measurements to describe them. A nanometer (nm) is one
billionth of a meter, and is an appropriately sized unit for this
use. Using this terminology, then, the portion of the electromagnetic
energy spectrum which our eye and brain interpret as light, extends
from approximately 700 nm on the long (red) end to about 400 nm
on the short (violet) end.
Visible Light Spectrum (nm)
:
- 700
- 630 = red
- 630
- 590 = orange
- 590
- 550 = yellow
- 550
- 490 = green
- 490
- 440 = blue
- 440
- 400 = violet
(For
generations, students have been introduced to "Mr. Roy G. Biv",
as a simple device for remembering the order of the colors in the
light spectrum). Not to get too far afield from our subject matter,
it is necessary to mention that this spectrum extends greatly on
either side of the narrow visible range: into ultraviolet, xrays
and gamma rays on the short end, and into infrared, microwaves and
radiowaves on the long end. The little segment of it that we are
concerned with in this class, not only powers vision, but also photosynthesis,
and many other biologically relevant processes. It is also important
to point out that the energy content of the various colors of light
is related, in an inverse way, to their wavelength. That is, light
of shorter wavelength is more energetic than light of longer wavelength.
Selective
Absorption: The color of most objects, gems included, is a result
of a process called "selective absorption". Let's take
an example: suppose you have on a yellow shirt, Why is it yellow?
The fibers and dyes in it absorb only some of the wavelengths of
the white light that hits them, primarily in the red, orange, green,
blue and violet bands. The wavelengths that are left (the yellow
ones) are reflected back to the eye of the observer whose brain
interprets light energy of that wavelength, as what we call yellow.
I'm sure you can see how a shirt could be greenish yellow or orangey
yellow if wavelengths slightly shorter or longer than yellow were
also reflected, and red or blue if it had a quite different pattern
of selective absorption. With opaque objects it is the color of
reflected light that we see, with transparent and translucent ones,
the color we see consists of a mix of both their reflected and transmitted
wavelengths.
Let's see if we can put together the information on transparency
with that on color :
- Transparency
will depend on the relative proportion of light reflected, transmitted
and absorbed by a gem. The color of the gem will depend on what
is reflected or transmitted after selective absorption has removed
some portion of the spectrum.
- If
none of the wavelengths are absorbed: the gem will be colorless
if it is transparent, or white if opaque.
- If
equal amounts of each wavelength are absorbed: the gem will
be grey.
- If
all wavelengths are absorbed equally and completely: the
gem will be black.
- In
colored gems : we will see a mix of wavelengths which were
not absorbed and which (depending on reflectance vs transmittance)
will give us a colored tranparent, translucent or opaque
gem.
Ok,
so selective absorption determines color, but what, then, determines
selective absorption, you ask? That is, why, precisely, do rubies
look red and sapphires look blue? The basic answer is simple, and
two-fold, and goes right back to the basic point previously made
in Lesson 3 regarding all gem properties. Selective absorption in
gems is determined by an interplay between their chemical makeup,
and their three dimensional structure.
The atoms (or ions) which create color in a gem are called "chromophores".
Some of the most common chromophores in gemstones are: atoms of
titanium, vanadium, chromium, manganese, iron, cobalt, nickel, copper,
nitrogen, and boron and their various ions.
(A new, undefined term, "ion", has crept in here, so let's
deal with that). Atoms are made of smaller particles: protons, neutrons
and electrons. The protons have a positive charge (+) and the electrons
a negative one (-). In an atom, such as oxygen, or iron, or any
other, the number of protons and electrons are equal making it,
overall, a neutral body.
Suffice it to say, that chemical and physical events can, and do,
occur that add or subtract electrons from atoms, making them into
negatively or positively charged bodies called ions. Fe is the chemical
symbol for a neutral iron atom, Fe+2 designates
an iron ion (an atom of iron which has lost two of its electrons),
Fe+3 has lost three, Cl- is a chlorine atom that has
gained an electron, etc. The main point for you to understand is
that events that occur in the "life" of gems and minerals
(or in a gem enhancer's laboratory) can change the ionic state of
their constituent atoms and ions, and thereby affect their color.
Back to the main point, the presence of various chromophores, as
well as certain details of the three dimensional structure of the
material itself, cause the selective absorption, which, in turn,
causes color. To put it another way, both the presence of particular
atoms and ions, as well as specific crytal "defects" such
as missing atoms or extra ones, areas of compression or strain,
can act as the agents of color in gems.
Idiochromatic vs Allochromatic Gems :
With
regard to the source of their color, gems fall into two categories:
idiochromatic and allochromatic. Idiochromatic gems derive their
color simply from the chemistry of their basic formula. Due to this
fact, such gems will always occur in various shades of the same
basic color. The other group (more common) are allochromatic, meaning
that the chemistry of their basic formula does not cause any selective
absorption so in the pure state, they are white or colorless. In
gems of this sort it is tiny, trace amounts of impurities that act
as the chromophores. Such gems occur in colorless forms as well
as in a variety of other colors depending on the nature and amount
of the "contaminants" in them.
[I think you'd probably get an argument from someone who is admiring
their beautiful blue sapphire, if you called the tiny amounts of
titanium and iron that give it that color,"contaminants",
though.]
Some
examples of idiochromatic gems are: peridot containing iron, (Fe),
rhodochrosite with manganese (Mn) and cuprite and malachite containing
copper (Cu).
Idiochromatic
Gems :
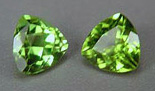
Peridot (Fe+2)
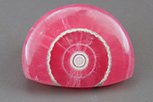
Rhodocrosite
(Mn)
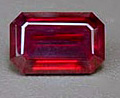
Cuprite
(Cu+1)
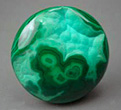
Malachite
(Cu+2)
Hey
wait a minute, you say cuprite is red, malachite is green, and
both contain copper! What gives? Welcome to the wonderful world
of gem color! It is not quite as simple as: this element makes
this color, and that element makes another color. Each gem's color
is determined by an interplay between its chemical makeup (including
the ionic state of its chromophores) and its structure.
To
further pursue this point: some emeralds are green due to chromium
content, while some get their green color from vanadium. So, iron
(as in peridot), copper, chromium or vanadium can each be responsible
for "greenness" in a gem. But on the other hand, chromium
in corundum makes red rubies, and iron in chalcedony, makes orangey
carnelian, but in sapphires gives us yellow. Futhermore, green zircons
and green diamonds get their color not from chromophores, but from
crystal defects.
Allochromatic
Gems :
Some
examples of allochromatic gems are: beryl, corundum, quartz, grossular
garnet, tourmaline, topaz, spinel and nephrite jade. In some cases
the "pure" material is the most common and therefore the
lowest in value (corundum, quartz, beryl and topaz are in this category);
but in others, the pure form is so rare as to be a high value collector's
item. This is especially true in the case of grossular garnet, tourmaline
and nephrite jade. Colorless spinel is so rare that it literally
has not been found in Nature; we know it can exist, though, because
colorless synthetic spinel is made in labs.
A
good example of an allochromatic gem species is corundum. Pure Al2O3
is colorless, as in white sapphire, but if we add just a tiny bit
of iron to the mix then we get yellow or orange fancy sapphire,
pair the iron with a bit of titanium, and the gem is the familiar
blue, and if chromium is the chromophore, then the corundum is red
and called ruby.
Allochromatic
Gems (in their pure state) :
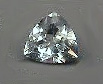
Colorless
Beryl (Goshenite)
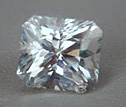
"White"
Sapphire
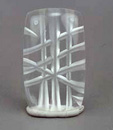
Colorless
Quartz (rock crystal)
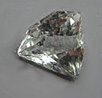
Colorless
Grossular Garnet (leucogarnet)
Allochromatic
Gems (in their impure state) :
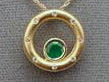
Beryl
: Emerald (chromium or vanadium)

Corundum
: Sapphire (titanium and iron)
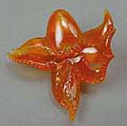
Quartz
: Carnelian (iron)
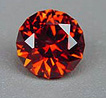
Garnet
: Spessartite (manganese)
Other
Sources of Color :
Some
gems get their color (or apparent color) from visible to microscopic
inclusions of other minerals within them. One of the most beautiful
of all the chalcedonies, often called "gem silica" but
more properly termed "chrysocolla chalcedony" has a vivid
blue-green color. The minute quartz crystals are actually colorless,
but in amongst them are tiny crystals of the blue green (very soft)
mineral, chrysocolla. The overall impression, in the best specimens,
is a translucent chrysocolla colored gem, with the durabililty of
quartz.
In the varieties known variously as strawberry and raspberry quartzes,
visible particles of red or red-orange hematite in the colorless
quartz, create a pink, orangey, red or polkadot looking gem, depending
on their size and number.
Inclusions
:
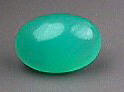
Chrysocolla
Quartz
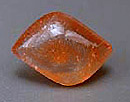
Strawberry
Quartz
Patterns
in color : banding/zoning :
One
of the most common features of some of the aggregate gems is the
presence of patterning. Since these gems are formed from very tiny
single crystals, we can easily envision conditions where differently
colored pools or batches of tiny crystals mix and intermesh creating
bands, dots or other patterns. Agates and jaspers are the most commonly
seen gems with strong patterns.
It
frequently happens that single crystal gems subjected to changing
conditions during their growth can also show bands or zones of different
colors or shades of the same color. When these are dramatic and
attractive, they are desirable, but far more commonly, gems of this
type have nondescript, patchy, or zoned coloration, and are considered
inferior to more evenly colored pieces.
Aggregates
With Patterns :
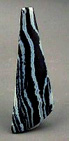
Zebra
Agate
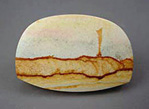
Picture
Jasper
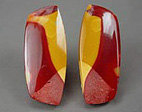
Mookaite
Jasper
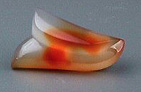
Carnelian
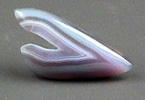
lavendar
Agate
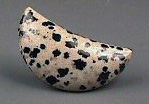
Dalmation
Jasper
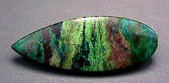
Rain
Forest Jasper
Single
Crystal Gems with Attractive Color Zoning :
Ametrine
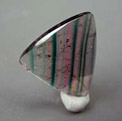
Multi-color
Tourmaline
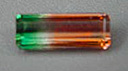
Watermelon
Tourmaline
Color
Descriptions in Colored Gemstones :
There
are three aspects to a formal colored stone color description: hue,
tone, and saturation. Using these three descriptors, very detailed
and nuanced color discriminations can be made, and communicated,
between gemologists, jewelers and gem buyers. Let's take them each
in turn :
Hue :
The
hue of a gem is its basic position in the color spectrum: red, orange,
yellow, green, blue or violet but it also includes all the possible
intermediates like slightly yellowish orange, or moderately bluish
green.
Tone :
The
tone of a gem, basically how light or dark the color, is independent
of its hue and ranges from so light as to appear virtually colorless,
to so dark as to look black.
Saturation :
The
least commonly quantified aspect of gem color is "saturation",
which is a measure of the purity of color, that is, the relative
presence or absense of modifying grey or brown hues. It turns out
that in most cases, as long as the hue and tone are reasonably nice,
it is the degree of saturation of color that is the prime value
setter in gemstones.
You
might think as to why does color description need to be so formalized?
The main reasons are listed below :
- Small
color differences mean big dollars!: in the rarified world of
gem and jewelry connoisseurs, zeros can be added to prices based
on what look like small differences in color to the rest of
us.
- Commonly
used adjectives are subjective, and culturally based: Without
some system of regularizing color descriptions it is very difficult
to communicate color information efficiently.
- For
example: Give three of your friends a unlabeled color chart
and ask them to show you "royal blue" or "lime green", or
medium orange. I can guarantee you'll get three noticeably
different color choices from each of them. Additionally,
limes may not be a familiar fruit in the country where you
wish to purchase a gem, or royalty there may be associated
with yellow, not blue.
- Color
memory is notoriously unreliable. Without a system whereby precise
color coordinates can be recorded, there is little chance of
doing a good job matching a new piece to an existing one.
- A couple
of simple exercises can verify this statement: You have
a nice sunny yellow paint in the kitchen, but some of it
is chipped off and needs repair. You go to the paint store
and pick the color from the paint charts that matches the
color in your memory what are the chances it will, in fact,
match? Or, let's say you want to buy a new shirt to match
your favorite brown pants: good luck, if you don't wear
or take those pants with you when you go shirt shopping!
GIA
Color Description/Grading System :
One well done, and widely used, system for color description
is that developed and taught by GIA (Gemological Institute of America).
Although not universal, it is familiar world-wide, and the basis
for most formal gem description and evaluation in the US and Europe.
Since
the wavelengths and light colors grade into one another in infinitesimal
changes, there are an essentially infinite number of hues which
could potentially be described. Most of these hues would be indistinguishable
from each other to our eyes, so GIA has settled on a group of 31
which humans with normal color vision (and some training) can discriminate.
The set of plastic gem models below is a representation of those
31. (It should be noted that GIA has taken some liberties with the
traditional "Roy G. Biv" spectral colors, deleting indigo,
and adding purple after violet. In the set below, then, you see:
red, orange, yellow, green, blue, violet and purple. The intermediates
are described by terms like slightly, moderately and strongly to
indicate a spectral hue modified to various degrees by those on
either side of it on the spectrum. It also recognizes hues which
are exactly 50/50 mixes such as red-orange and blue-green.
HUE
:
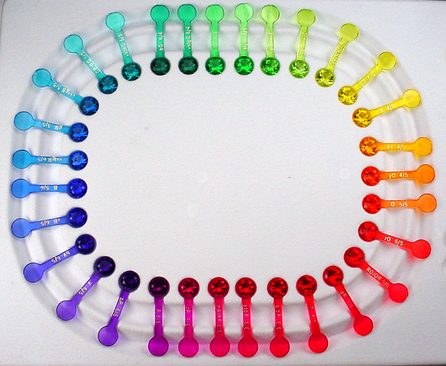
Gia's
31 basic gem hues
The
hue, then, consists of the key (or spectral) color plus adjectives
describing the hue and strength of the secondary modifying color,
if any. For example: slightly purplish red, symbolized by "sl
p R" (sl for degree, lowercase p for the secondary hue (purple),
and upper case R for the primary hue, (red). The description strongly
yellowish green: "st y G" would be decoded using the same
logic. Once you have been trained to see nuances of color, you recognize
that pure spectral colors in gems are quite rare, and as a result,
costly. For example: a hue of simply "B" would be pure
spectral blue and if other factors of color and clarity were good,
the piece would command a premium price.
If you are thinking that it looks like there are more gradations
in the blues and greens in the display above, than in the other
colors, you are right. Human vision has finer powers of color discrimination
in that part of the spectrum, which this system takes into account.
TONE
:
Each
of the 31 hues exists in a range of tones from almost colorless
to almost black. GIA labels the tones as 0 - 10. {0 ( appears colorless),
1 (extremely light,) 2 (very light), 3 (light), 4 (medium light),
5 (medium), 6 (medium dark), 7 (dark), 8 (very dark), 9 (extremely
dark), 10 (appears black).
The
figure below represents the 2-8 part of that range, which is, in
the great majority of cases, the range for marketable colored gems.
For most species the most valuable tones are in the 5-6 range. The
set below is shown without hue, and it takes practice and patience
for the would-be colored gem grader to learn to superimpose hue
onto these, and get a valid tone reading.
An
additional complication comes from the fact that gem species differ
in their inherent tone ranges. For example, let's compare an aquamarine
and a pyrope garnet each of tone 6. Objectively, each is exactly
the same, but that depth of color is about the deepest that will
ever be found for aquamarine and the about lightest possible for
any pyrope. One should not be surprised, then, to find the aqua
dealer calling her stone "very dark" and the garnet seller
raving about how beautifully light his stone is when they are both
"6"'s.
GIA's
tone scale from 2 (very light) to 8 (very dark)
Finally,
it's time to examine the most subtle aspect of gem color, saturation:
in a manner of speaking, this measure is the degree to which the
other spectral colors "muddy up" the main hue. Think of
a can of pure red paint and start adding in various amounts of all
the other colors the more you add of the other spectral hues, the
"browner" the red will get. Now do the same thing with
a can of pure blue: the more you add the "greyer" the
blue will get. In general, desaturating "warm colors"
makes them look brownish while the same effect in "cool"
colors looks more grey. Therefore, GIA's system of describing saturation
makes a distinction between cool and warm hues.
Warm
hues = green through red (desaturated to brown) Cool hues = purple
through blue (desaturated to grey)
Six
degrees are recognized ranging from : 1 (brownish/greyish), 2 (slightly
brownish/greyish), 3 (very slightly brownish/greyish), 4 (moderately
strong), 5 (strong), 6 (vivid)
In the figures below, you can get a better idea of the saturation
effect by looking at the flat end of the plastic gem replica,
rather than the "gem part".
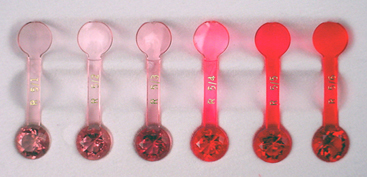
GIA's
six degrees of warm hue saturation
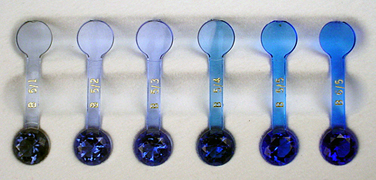
GIA's
six degrees of cool hue saturation
When
giving a gem's formal color description in words, then, the gem
below might be said to be: medium dark, slightly greyish, blue-violet.
It sounds more natural to put the tone, saturation and hue in
that order. In a numeric description as required in offical gem
grading documents, however: the order would be: hue, tone and
saturation, thus: BV 6/2.
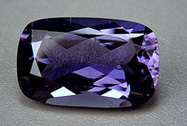
Iolite
: In words : Medium dark, slightly greyish, blue-violet = official
"grade" : BV 6/2
One
final point on the GIA color grading scheme: Two non-spectral
colors are used (in addition to the officially sanctioned 31)
and those are pink (pk) and brown (br). If one were to strictly
follow the GIA system, all shades of pink are really lighter tones
of red, and brown is simply desaturated orange. It is rather a
matter of bowing to tradition and convenience to recognize pink
and brown as "colors" in their own right. You will see
evidence of this practice in the color description below.
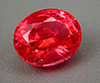
Spinel
: Medium Dark, Moderately Orangey, Strong Pink Mod O PK 6/5
Color
Grading in Diamonds :
You
will recall from Lesson 1 that within the gem industry, there are
separate systems for marketing, grading, and describing colored
gemstones and diamonds. For virtually all natural diamonds, discernable
color is a negative attribute. The closer it is to an absolutely
colorless condition, the more highly valued is the gem.
In
the case of what are called "fancy" diamonds, whose color
is both intense enough, and attractive enough, to be desirable,
color is described and evaluated in a similar manner to that used
for colored stones. There is sort of a "U" shaped value
curve for diamonds, whereby the highest values accrue to only the
whitest, and then, again, to the most vividly colored specimens,
with value bottoming out in the central ranges where there is just
a bit, to a moderate amount, of color.
Although
most of the diamonds you might see on a day-to-day basis are called
"white" and appear so, a little study and comparison will
verify that a truly colorless diamond is a thing of great rarity,
and the vast majority of diamond gems are actually tinted with small
but noticeable amounts of yellow or brown.
I was taking some liberties, perhaps, by using the GIA system in
the preceding discussion of colored gem descriptions, but without
doubt, the GIA system is the one to learn if you are interested
in diamond colors and value. It is understood everywhere in the
world, and used for formal grading in most countries. Even competing
gem grading laboratories either use the GIA system, or provide a
key to translate theirs to it. For example AGS, The American Gem
Society uses 0 - 10 for their color grading scale (0 = D, 0.5 =
E, 9.5 = W, 10 = X - Z, etc.), but gives the customer an exact conversion
scale to the GIA system with their reports.
Before
the GIA system was developed (beginning in the 1930's), there were
as many diamond color descriptions as there were diamond sellers.
Many of them used A, B, C and A+, AA, AAA etc. while others used
adjectives like "river" and "cape". It is easy
to see how difficult it would be to have a reliable system for trade
under those conditions. GIA's scale did away with A, B and C because
of their long histories and diverse useages, and developed a system
based on color grades from D-Z for "colorless" stones,
plus the term "fancy" to indicate those whose strong color
made them more, rather than less, valuable.
What
the Letters Mean :
D, E, F :
Gems
in this range appear colorless even in larger sizes, only a highly
trained diamond grader can tell the differences between them.
G,
H, I :
These
grades describe gems that look colorless to most viewers in smaller
sizes and if mounted.
J,
K, L :
Small
and mounted stones of these grades look near colorless, but larger
and unset gems begin to have noticeable color
M-Z
:
Gems
in this range are worth much less than higher color grades and range
from some color noticeable to distinctly light yellow (or brown).
Z+
:
Beyond
Z is the range of the "fancy" diamonds whose value is
based on their hue tone and saturation, as in colored stones. In
general browns are least valuable with yellow, orange, and green
worth considerably more. The pinnacle of value for naturally colored
diamonds is occupied by purple, blue, pink, and at the very tip-top,
red.
The images below may or may not be enlightening, based on the
characteristics of your vision, viewing circumstances, and monitor
calibration, but they will hopefully serve to illustrate at least
some of the aspects of our topic.

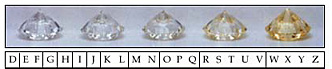
How
Do they Do That ?
After
straining your eyes to see the minute changes in the illustrations
above, you might ask, how can a grader do it, especially when a
great deal of money rides on the difference between, say an F and
a G grade, or an L and an M? I might joke that the answer is "verrrry,
carefully". In actuality, not everyone can become a successful
diamond grader there is both exacting training and "raw talent"
involved. In practical terms, the mechanics of the process is that
the stones to be color graded are serially compared to the gems
in a special "master stone" set, under controlled conditions
of lighting and viewing.
Gemology
Then and Now :
Pre-modern
:
What
we could refer to as modern-style gemology began and developed in
the period between 1930 to 1950. Prior to that time gemology was
a simpler discipline to master and practice than it is today. The
mains reasons for this are :
-
Relatively
few gem species and mining locales were known. Although gem
exploration was certainly engaged in, the scale was much more
limited, and as a result there were but a few dozen known
species and varieties of gems, and a correspondingly small
set of mine sites.
-
The
tools of the trade were limited. A magnifier, a hardness testers,
some acid for detecting carbonates, and balances for determining
specific gravity were all that was in the gemologists' kit.
We might say that practicioners of earlier eras were "optical
gemologists". Most of the gems' measurable properties were
either physical (density, hardness, stability, crystal habit)
or those which could be seen with the naked eye, such as,
luster and color.
-
Relatively
few enhancement processes existed. Heating, dyeing and coating
were long-familiar techniques which for the most part were
easy for a trained eye to detect.
-
Synthetics
and simulants were few. Only a handful of gems had been synthesized
prior to 1950 and those had clear cut signs indicating their
"non-natural" origin. Simulants were common, but they were
mostly either assembled gems, or natural substitutes, which,
again, were relatively easy to detect with the knowledge and
equipment of the day.
To give an
example, I'll relate a true story: Recently, a client came to
a gemologist I know, with an antique ring that they had inherited.
Along with the ring was a jeweler's certificate, dated 1870, certifying
that the gem was an emerald. A variety of tests soon revealed
that the stone was, in fact, a green tourmaline. Had the client's
ancestor been duped by an unscrupulous jeweler? Most likely not.
With the tools and knowledge available in the 1870's, a green
stone of hardness greater than 7, and with a vitreous luster that
came from South America (as this one did) would have been called
an emerald. Thus it is, that a lot of the "rubies" in museum collections
are actually red spinels, and much of the "jade" pieces among
the displayed artifacts are serpentine or hydrogrossular garnet.
Gemology
Today :
Today's
gemology is a very different sort of game I say "game"
because it has quite literally become a tug of war, or an "arms
race", if you will, between gemologists and those who seek
to profit from misinformation or ignorance. In comparison with the
list above we find:
-
There
are now hundreds of recognized gem varieties and species, and
the rate of discovery of them is increasing. The world's hunger,
and willingness to pay dearly, for gems has fueled an unprecidented
rate of exploration for new sources of both colored stones and
diamonds.
As
an example: a new species in the beryl group of gems was identified
in 2002. The discovery of new varieties, and new sources for
known varieties, happens very frequently, but a completely unknown
species of gem mineral, is as big a piece of news
to the gemological/mineralogical world as a new species of mammal
or bird would be to the world of biology.
Pezzottaite
a new cesium rich species of Beryl, discovered in Madagascar,
Cat's Eye Stone
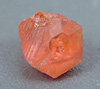
Uncut
Crystal
- It is
very common for different mine sites with different geological
histories to yield the same variety of gem, but with slightly
different chemistries and inclusions. Because identification
often depends on subtle nuances of inclusions and/or chemistry,
the definitions and identification criteria must constantly
be modified to reflect and incorporate these new discoveries.
To give
a fairly dramatic example: until the 1960's an emerald,
by definition, was beryl of medium to medium dark, strong
to vivid slightly bluish to slightly yellowish green, colored
by the element chromium. The discovery of African beryls
which looked like emeralds but contained vanadium rather
than chromium, led to vigorous (some would say bloody) debate,
that culminated in changing the official definition of an
emerald (to include vanadium as a possible chromophore).
It also made the use of a standard gemological tool, the
Chelsea filter, which reveals the presence of chromium,
pretty much obsolete for its, then, major purpose of separating
emerald from most of its simulants.
- Enhancements
have become huge business, and the temptation NOT to disclose
them is as large as ever. Very sophisticated techniques of
heating, dyeing, coating, stabilizing and irradiating have
been, and are being, developed. These require ever more knowledge,
and equipment on the part of the gemologist or gemological
laboratory.
The
best recent example of this is the sudden appearance on
the market around the year 2000 of an abnormal quantity
of the rare "padparashah" variety of fancy sapphire (its
color is pink/orange). Initially, collectors rushed to
grab the abundance, as such opportunities are generally
very transitory. The enriched supply continued, though,
and increased raising the suspicions of gem dealers and
gemologists world wide, and sending them scurrying to
the field, and to their laboratories. It turns out that
a brand new, very difficult to detect, type of enhancement,
called "beryllium diffusion" was the culprit. The news
stunned the gem world in 2002, and made the gem treaters
who sold these bogus stones wealthy, in the short period
before they were found out.
Beryllium
diffused, enhanced "padparashah" sapphire
- Last,
but to be sure, one of the most important new developments,
has been the introduction of multiple new technologies for
synthesizing gems. The easy to detect early methods are still
being used, but an increasing percentage of high (and even
lower) value gems are being synthesized by methods that so
closely simulate natural formation conditions, that the gems
they produce require extreme vigilance, and ever more sophisticated
analyses to reveal.
Thus, today's
gemologists, as much as those in any other rapidly advancing area
of science, must constantly watch the literature, and keep themselves
abreast of any new tools or techniques which they can use to keep
"one step ahead" of those who would deceive. As we turn our attention
to laboratory gemology, we'll be looking at several basic properties
or "behaviors" of light and seeing how each, in turn, is useful
in describing gemstones. The aim of this part of the lesson is
to acquaint you with first, the basic facts of these properties,
and subsequently show how they are measured, and/or how they can
be used to solve problems in gem identity.
Behavior of Light 1 : Refraction
We all know
that the "speed of light" is the stuff of which cosmic
measurements are made, and you may or may not have that famous
figure in your store of readily retrieved facts. When we discuss
the speed of light in an astronomical context we are thinking
of the speed of light in a vacuum (the absense of matter), but
light slows down when it travels through any medium denser than
a vacuum.
Air is not
a vacuum, so light slows as it enters our atmosphere from space,
in the same way, light slows from its "air speed" when
it enters any material of greater density than air, which would
include all gemstones. If a light ray enters the gem at any angle,
other than directly perpendicular, to a surface, then it also
bends as it slows. The degree of slowing is determined by the
density of gem, and the degree of bending is determined both by
density and by the angle of its entry.
The ratio
of the speed of light in air, to the speed of light in a gem,
is called the gem's refractive index or RI. It differs, sometimes
dramatically, between gem species, and is one of the most useful
gem identification criteria. In natural gems it ranges from
about 1.2 to 2.6. and can, in most cases, be measured by an
instrument called a refractometer.
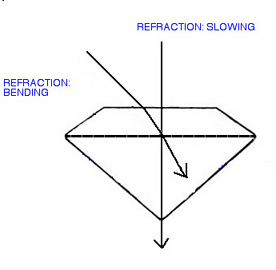
Refraction
includes the slowing and the (usually) consequent bending of light
as it enters a gem
Behavior
of Light 2 : Dispersion
Dispersion
(sometimes called "fire"), is the separation of white
light into its spectral colors. It may be observed as specks of
red, blue or green which flicker as the gem is turned.
The cause
of this phenomenon is the differential refraction (bending) of
the various wavelengths of light as they travel through a gem.
Red (long wavelength) bends less, violet (shorter wavelength)
bends more. This causes the colors to become separated. Although
dispersion, theoretically, occurs in all gems, the degree depends
on the RI of the gem material, and only those gems with sufficiently
high RIs, have dispersion which is pronounced enough to be actually
visible.
The exact
figures for dispersion can be painstakingly measured in a laboratory
setting using special equipment, and then calculated as the difference
between the RIs of red light and violet light in a given species.
Potential dispersion in gems, thusly measured, ranges from .007
to .280.
Outside
the lab, dispersion is generally judged visually, without instruments,
simply as: absent, slight, moderate, strong or very strong.
The degree of visible dispersion is affected by species (due
to RI), but also by the body color, and cut proportions of the
gem.
In general,
the denser the gem, the greater its potential dispersion. Light
body color, and steep crown angles enhance the display, whereas
dark body color and shallow crown angles diminish it.
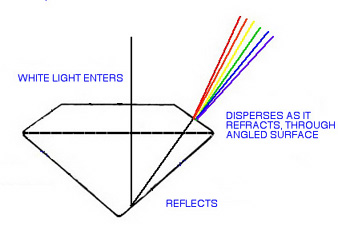
Dispersion
of white light as it leaves a gem
Examples
of gems with slight dispersion potential are : fluorite (.007),
common glass ("crown" or silica glass) (.010), and quartz
(.013). Regardless of color or cut, these gems just aren't going
to show visible dispersion, the effect is too slight.
Those with
moderate potential for dispersion include: tourmaline (.017),
corundum (.018) and spinel (.020). Such gems rarely show visible
dispersion, but an occasional light colored specimen of substantial
size with very high crown angles may do so.
Examples
of strongly dispersive gems are: zircon (.038), diamond and Benitoite
( both .044), and cubic zirconia (a synthetic), (.066). Gems in
this range will usually show dispersion. Exceptions might be those
of very dark body color, or small pieces cut with rather low crown
angles.
Very strongly
dispersive gems include: sphalerite (.156), strontium titanite
(a synthetic) (.190) and synthetic rutile (.280). There would
be very few cases where a gem in this group did not show substantial
dispersion.
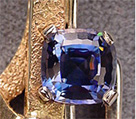
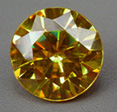
Benitoite
and sphalerite both with cuts and body colors which permit their
substantial dispersion to show
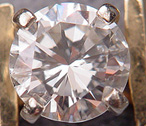
Diamond
a gem admired for its dispersion
Diamond is
the most well known gem that shows dispersion, and it is one of
that gem's most appealing attributes. The success of either a
natural or man-made diamond simulant, depends to a large extent
on how well the substitute matches diamond in this characteristic.
Before the
post-1950's crop of synthetic diamond simulants came on the market,
the choices were limited to glass, foil-backed glass, or amongst
crystalline gems: white sapphire, white beryl, white topaz or
white zircon. Of that group, zircon made the best simulant due
to its dispersion being much closer to that of diamond than any
of the others.
Quite a few
synthetics have been created since then, but, only one, like the
Baby Bear's porridge, is "just right" and that is cubic
zirconia. Even though its dispersion figures are a bit too high,
in the small sizes usually encountered, the difference is not
obvious. YAG on the other hand is without noticeable dispersion
and looks very "glassy", while synthetic rutile and
strontium titanite have way too much to look convincing.
The first
set of pictures below shows, respectively, the two most convincing
natural and man-made simulants. The second set shows a group
of three temporarily popular, but unconvincing synthetics.
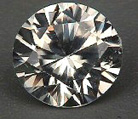
White
Zircon (natural)
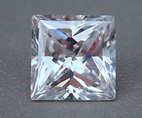
Synthetic
Cubic Zirconia
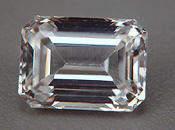
YAG
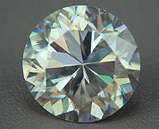
Sythetic
Rutile (less)
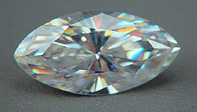
Sythetic
Strontium Titanite (more)
Behavior
of Light 3 : Light is Influenced by the Optic Character of a Gem
There are
two groups of gems with regard to light refraction, each is said
to have a different "optic character": SR or DR.
SR
stands for singly refractive. In such gems, each beam of light
entering the gem stays as a single beam which has a single refractive
index (travels at the same speed), regardless of the direction
from which it enters. In this group we find all amorphous gem
materials, such as opal, glass, amber, etc. as well as all crystalline
gems belonging to the cubic (isometric) system. The most commonly
encountered gems of the cubic system are: diamond, garnet and
spinel.
Gems whose optic charcter is SR :
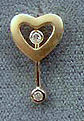
Diamond
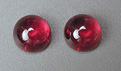
Garnet
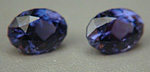
Spinel
(cubic system)
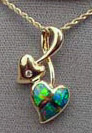
Opal
(amorphous)
DR
stands for doubly refractive. In such gems, single beams of light
upon entering the gem, are split into two separate beams, which
then travel perpendicularly to each other. Each of the resultant
beams takes a different path through the crystal and, consequently,
has its own speed. Such gems, then, have two RIs, one for each
half of the original beam. In this group are all the gems of the
non-cubic crystal systems.
DR Gems :
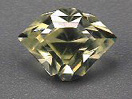
Amblygonite
(triclinic)
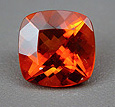
Citrine Quartz (trigonal)
Pearl
(orthorhombic)
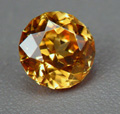
Scheelite
(tetragonal)
Important
Note :
Each DR gem,
based on details of its crystal structure, has either one or two
directions in which the light entering it behaves as if the gem
is SR. These directions are known as "optic axes". Those
species with a single optic axis are known as "uniaxial"
and those with two are, logically, called "biaxial".
It is sufficient to simply note this at present, but we will return
to this fact and see that the existence of optic axes can constrain
the methods by which we test some of the optical properties of
gems, as well as serve as a valuable identification criterion
in its own right.
Birefringence
:
BR
:
Birefringence,
a property of DR gems only, is measured as the difference between
the high and low RIs of the split beams. It ranges from a low
of .003 to a high of .287.
When a transparent
gem with high BR is faceted, and the view through the table direction
of that gem is not in an optic axis direction, the slightly "out
of sync" light beams can create an appearance of interior
"fuzziness" or in larger stones, can show up as two
distinct images of each facet edge. This is known as "facet
doubling" and it can be a pain in the neck to a facetor who,
in trying to prevent it, must find an optic axis direction for
the table of the stone. But it can also be a valuable identifying
characteristic that can often be seen with the naked eye or a
simple 10x loupe.
For reasons
unknown to me (perhaps it is a "British-ism"), the
Hall text abbreviates birefrigence as "DR" in the
tables at the back of the book and on the individual species
pages. BR as used in this lecture, is the standard abbreviation
used in most books.
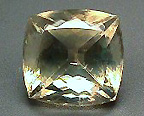
Calcite
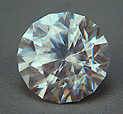
Synthetic Moissanite
Gems with high BR, showing facet doubling
The pictures below demonstrate the effect
very clearly. The material below has been cut into a geometric
form known as a "cuboctahedron" from synthetic rutile
(which has extreme birefringence = .287). It has been placed
above a single black ink dot on the surface. The left photo
shows the view through the central face which has been cut
on an optic axis. Note that the dot is clearly visible as
"one" as are the reflections of its image in the
other faces. In the second photo the piece of rutile has been
turned so that we view the dot through another of its faces
(one not on an optic axis). Now we see two images of the dot.
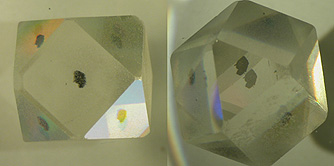
Synthetic
rutile above a single black dot : viewed in the optic axis
direction, viewed in a non-optic axis direction
Note :
The
newest diamond simulant on the market is called Moissanite.
(That's the one that required the new generation of electrical
conductivity testers). Fact : Moissanite is markedly birefringent;
diamond, being SR, has no birefrigence.
Behavior
of Light 4 : Pleochroism
As
we know, DR gems split light into two perpendicular rays, each
taking different paths through the crystal : one ramification
of this is birefringence, another is pleochroism. Pleochroism
is the property of DR gems which results in their showing different
colors, or different shades of the same color, when viewed in
different crystal axis directions.
How
can this be? Think again of the crystal lattice of a DR gem,
made of carefully laid out atoms of the gem's component elements
(and the trace chromophore elements), with fixed distances and
densities that can vary depending on direction. If two beams
of light take a different path through this lattice, they may
then be affected differently by selective absorption and emerge
with different colors.
This
effect can be weak, moderate or strong, depending on the species
of gem, the colors involved, and also on the color tone of the
particular piece. A very light piece of a pleochroic species
will show the effect less clearly than a more richly colored
one. Unless the effect is extreme (as it is in iolite and Andalusite),
we generally do not see it in a cut gem, because the bouncing
and mixing of the light caused by the internal reflections from
facets and edges blends the colors together and obscures it.
Dichroic gems (like corundum) show two different colors while
trichroic gems (like iolite) show three.
Pleochroism will not be observed in SR gems, nor in DR gems
when looking through an optic axis direction.
In
most cases, pleochroism can best be observed using an instrument
called a dichroscope. This cleverly made little tool uses a
piece of highly birefringent colorless calcite to split the
incoming light into two beams which are bounced off tiny mirrors
positioned inside so as to reflect each of the two beams onto
a pair of side-by-side viewing windows. This placement allows
the viewer to simultaneously see light that has traveled two
different paths through the gem or crystal being viewed. Simultaneous
viewing isn't absolutely necessary, but considering that these
effects are often subtle and color memory is poor, it is certainly
easier that way. All that is necessary to use this instrument
is a good light source, and a transparent to translucent gem.
Below
you see a dichroscope (about 2" long) and a simulated view
of a ruby gemstone, as it would appear through the dichroscope.
For the sake of clarity I have emphasized the color differences,
but they are not quite so distinct in reality. Ruby has an orangey
red color axis, and a purplish red color axis.
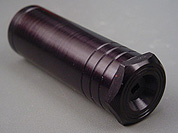
Calcite
Dichroscope
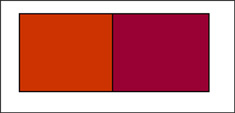
Simulated
view of ruby's characteristic pleochroism as it would appear
looking through a dichroscope
One
must view the gem being tested with the dichroscope from several
different directions because :
1. Some directions will be optic axis directions, in which there
would be no pleochroism shown, even if the gem were pleochroic.
So, if you based your conclusion on one direction only, there
would be a large chance for error.
2. Only two colors show at a time, so, although dichroism might
be detected from a single directional view, it would take more
than one to see all three colors in a trichroic stone.
When
strong, pleochroism may complicate the orientation process for
a cutter and/or create setting issues for a jeweler. The cutter
is going to want the most desirable or attractive color to be
that seen when looking through the gem's table. For example,
iolite has a lovely blue-violet axis, one that is grey, and
a third that is a near colorless light yellow. Few buyers are
interested in a grey or nearly colorless iolite.
A
large number of tourmaline stones have one axis that is an
opaque black! The other directions may show a lovely green
or pink, but if the gem is not cut so as to prevent light
bouncing from the black direction into the green or pink,
the color in the finished stone will look terrible--> a
muddy brown. To prevent this, a special "tourmaline cut"
has been devised whereby the sides of the offending axis are
cut so steep (approximately 70 degrees) that light from it
is prevented from reflecting back into the gem. This leaves
a gem with proportions that do not fit into standard prong
or bezel mounts. Jewelers have had to devise a special "tourmaline
mount" (as seen below) to accomodate such gems.
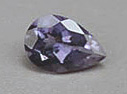
Iolite
showing a lot of its undesirable grey axis
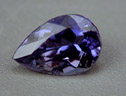
Iolite
oriented to show a near ideal blue-violet
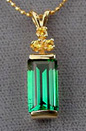
Tourmaline
cut gem mounted in "tourmaline mount"
Tanzanite
gems, which in the rough are trichroic, but after their standard
heating process become dichroic, have a blue and a purple axis.
Blue stones have a higher per carat value than purple ones,
but, unfortunately, the shape of this gem's crystals dictate
that the greatest yield comes from cutting a purple gem. The
cutter, then, must balance these two factors and try to orient
the stone so as to give the largest, best colored, and most
valuable stone from an individual piece of rough.
In
some gems, most notably Andalusite, all the colors are attractive
(brownish shades of green, red and yellow) and the mix of
them in the finished gem is considered desirable.
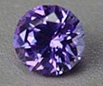
Tanzanite
with the purple color dominating

Tanzanite
with the blue color dominating
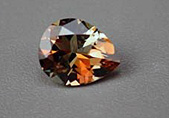
Andalusite
showing patches of all its colors
The
tools you have are limited to a dichroscope and a gem reference
book that lists the pleochroic colors of gems. You find that
iolite is trichroic, sapphire is dichroic and spinel, which
is SR, shows no pleochroism. You find that ruby is dichroic
and, garnet being cubic, and glass being amorphous, are SR and
have no dichroism. You have no other equipment.
Behavior of Light 5: Polarity of Light and the Optic
Character of Gems
The
rays of light from the environment or from standard man-made
sources are vibrating in all directions perpendicular to their
individual directions of propagation. They are said to be non-polarized.
With respect to this vibration, light is affected by the gem
materials it enters in two possible ways :
With
DR materials, each of the two light rays that result from the
splitting of an original beam, now vibrates in only one plane:
it is said to be "plane polarized". Light goes into
the gem non-polarized and comes out polarized. (The exception
is light that travels through such a gem in an optic axis direction.)
When we talk about such a pair of polarized rays, it is convenient
to call one the E/W ray (East/West) and the other the N/S ray
(North/South).
SR
materials have no such effect, the light remains non-polarized
as it travels through the gem. That is, light goes in and comes
out of the gem non-polarized. We can also picture this by saying
that light comes into the gem vibrating in all directions and
exits the same way.
This
property is one that is useful in gem identification and can
be readily detected with an instrument called a polariscope.
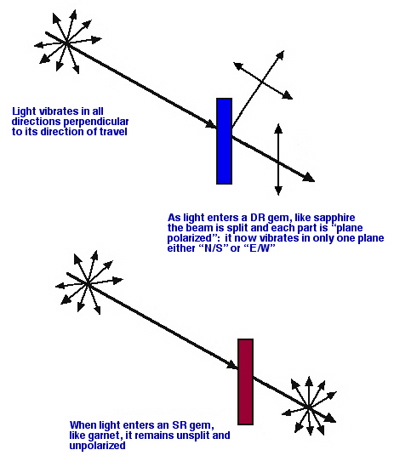
The
effect of optic character on the polarization of light
Brief
Review of SR -vs- DR Gems :
In singly refractive (SR) gems, light which enters remains as
non-polarized beams, and travels through all crystal directions
at the same speed. There is one refractive index (RI), no birefringence
and no pleochroism. The SR gems can be amorphous or those of
the cubic crystal system.
In doubly refractive (DR) gems, light which enters splits into
two perpendicular polarized beams. Each beam travels at its
own speed and has a separate RI which depends on direction.
Such gems have birefringence and may show pleochroism. A DR
gem can belong to any crystal system, other than cubic. All
DR gems have either one (uniaxial) or two (biaxial) optic axis
directions in which they will behave as SR.
Hint :
when
it comes to crystalline gems, if you commit diamond, garnet
and spinel to memory as SR, then virtually all other crystalline
gems you are likely to run into are DR. So, what is the optic
character of topaz? amethyst? enstatite? diopside? etc. etc.
Easy: Are they diamond, garnet or spinel No!, then they are
DR.
Testing for Optic Character :
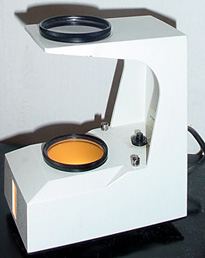
Polariscope
The
easiest way to test a gem for optic character is by using a
polariscope. It is composed of two polarizing lenses with a
light source below them. Each lens transmits only light that
vibrates in single plane, either N/S or E/W.
When
the light in the base is turned on, normal, unpolarized light
is produced, which becomes polarized, let's say N/S, as it
passes through the lower polarizer on the base of the unit.
The upper lens can be rotated freely. If the upper lens is
parallel to the lower one (also N/S), then the light travels
through it, and we see a lighted field. The picture below
is a photo taken looking into the upper lens as just described.
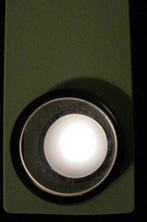
Polariscope in the "open filters" position
If
the upper lens is rotated 90 degrees, the N/S polarized light
passed by the lower lens is blocked by the, now, E/W position
of the upper lens. The view through a polariscope in this "crossed
filters" position is shown below :
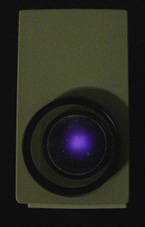
Polariscope
in the "crossed filters" position
The
DR Reaction :
Imagine taking a DR gem and placing it on top of the lower
lens when the filters are in the crossed position. Since a
DR gem actually functions as a small polarizing lens it now
becomes a, third or middle, polarizer. Each 90 degree turn
we give the gem changes the polarity of the light that goes
through it, E/W to N/S, and vice versa. With one 90 degree
turn, light that was blocked before can now get through, and
we see the gem brightly lit. Turn it 90 degrees more the stone
blinks dark as now it is producing light of the opposite polarization.
This reaction, dark, light, dark, light is that which is characteristic
of DR stones.
The
SR Reaction :
Much less impressive, but equally telling is the reaction of
an SR gem. Put an SR gem on the bottom filter (with the polariscope
in the crossed filters position) and it looks dark. Turn it
90 degrees still dark. No matter which direction you turn it,
it is always dark. This type of material does NOT act as a polarizing
lens and therefore doesn't change the polarity of the light
that goes through it.
It is logical for you to expect that since a polariscope tells
the optic character of a gem, it should show either a reaction
of either SR or DR when a gem is tested. Both SR and DR readings
are possible, as described above, but there is a also a third
possibility. Certain gems, when tested as described above, neither
blink dark and light, nor stay dark. They are light as intially
viewed and stay light no matter how they are turned! What is
going on? A gem with this reaction is an aggregate. (Remember,
an aggregate is a gem made up of microscopic to sub-microscopic
crystals all intermeshed together. Examples are various chalcedonies
and jade).
The
AGG Reaction :
All commonly used gem aggregates are DR, that is, the teeny
tiny little individual crystals making them up are DR. But these
little crystals are randomly oriented within the piece of material,
so that on average no matter which way you turn the piece, about
half of them are in their E/W position and half in their N/S
orientation. Thus, turning the gem has no effect, a good amount
of light is passed through it in any position. This reaction
is called AGG.
All
of the above is MUCH harder to put into words, or to read
and comprehend, than it is to observe and recognize. Let's
do some tests. In the picture below are three pieces of gem
material. One is quartz, one is glass and one is chalcedony.
We'll observe their reactions under the polariscope and see
what we can conclude.
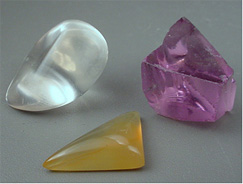
Items
to test : One is Chalcedony, one Glass and one Quartz
Specimen
1 :
The
first piece to be tested is the colorless one on the upper left.
See its reaction below: (Note that you can, by looking at its
shape, determine which of the three pieces it is, and that it
has been rotated approximately 90 degrees in the second picture.)
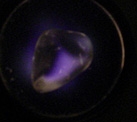
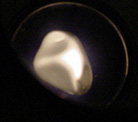
Specimen
1 : Under "crossed filters" in two positions
Specimen
2 :
The
next piece to be tested is the light purple one on the upper
right.
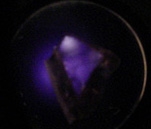
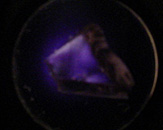
Specimen
2 : Under "crossed filters" in two positions
Specimen
3 :
The
final piece to be tested is the light brown one on the bottom.
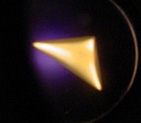
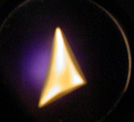
Specimen
3 : Under "crossed filters" in two positions
Keeping
in mind that glass is amorphous and therefore SR, quartz is
DR and chalcedony is a aggregate, it is quite clear which
is which.
Testing
Refractive Index :
The
refractive index (RI) of a gem is one of the most important
characteristics determining its appearance, and is also a very
useful piece of data for purposes of determining the species
of an unidentified gem.
Without Equipment :
Using
no equipment it is sometimes possible to determine an approximate
range for the RI of a polished gem. This is because RI generally
correlates physically with the density of a gem, and visually
with its luster, brilliance and dispersion. So, if I find a
gem which is heavy for its size, has a higher than vitreous
luster, is extremely brilliant and shows dispersion, I can just
about conclude that its RI will be found in the upper ranges
for gems. On the other hand, a notably lightweight piece with
sub-vitreous luster, little brilliance and no dispersion, is
probably to be found near the lower end of the RI range. I say
it is sometimes possible, though, because the degree of polish
or lack of it, the number and types of inclusions, and the color
or condition of the gem may make even a ballpark estimate difficult
without some kind of equipment.
Using Liquids of Known RI :
You
will recall from Lesson 3 how it is possible using liquids of
known specific gravity to work out an approximate SG for a gem
by immersing it and observing the reaction. There is a similar
technique that can be used for RI. This technique called "Relative
Refraction" uses liquids whose RIs are known. When a transparent
gem is immersed in a (colorless) liquid, how clearly we can
see details of the gem depends on the RI of the gem compared
to the RI of the liquid. A critical concept here is "relief";
we say that a gem has high relief when it stands out as sharply
visible in the liquid, and low relief when it doesn't. The greater
the RI of the gem above that of the liquid in which it is immersed,
the greater its relief.
1. A gem that is immersed in a liquid whose RI is well below
its own shows high relief, the gem and its details are clearly
visible
2. A gem that is immersed in a liquid whose RI is moderately
to slightly below its own shows moderate to slight relief, the
interior details and outline of the gem are harder to see.
3. A gem that is immersed in a liquid whose RI is the same as
or higher than its own shows no relief, no edge, or interior
detail is visible. The gem, if colored, looks simply like a
hazy colored area in the liquid, and if it is colorless, it
virtually seems to disappear!
Using this basic idea we can compare the relief of two different
gems in the same liquid, or we can test the same gem in different
liquids. Each technique would give us information about the
relative refractive index of the test pieces.
Three common liquids used in this sort of testing are: water
(RI = 1.33), a commercial compound called "Refractol"
(RI = 1.56) and methylene iodide (RI = 1.74).
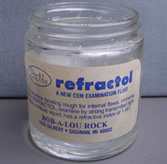
Liquid
sold commericially for relative refraction testing: RI = 1.56
Before
proceeding any further, let's see a demonstration: below is
a bottle of "Refractol" and the gem petalite. The
RI of petalite is between (1.50 - 1.51). Watch what happens
:
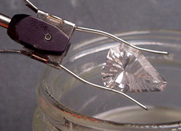
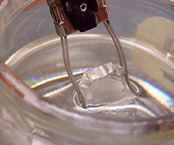
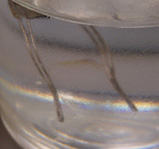
Going,
going, gone
Notice
the reflections and detail visible when the gem is in air, compared
to when it is immersed in the liquid. In the Refractol, it seems
to disappear. You can tell that there is something in the jaws
of the tweezers, but you can't make out any detail.
This
happened because the RI of the gem (1.50 - 1.51) was below that
of the liquid (1.56). If I had not already known that the gem
was petalite, but instead, was trying to identify an unknown,
this test would have eliminated a great many possibilities.
Another
reason why gems are sometimes immersed in such liquids is that
by diminishing the relief and reflections, internal characteristics
like color zoning, fractures and inclusions sometimes stand
out more clearly.
If you ever go to a store or show where gem rough is sold, you
can see potential purchasers dipping the rough into water the
dealer has provided to get a better interior view (or less appealingly,
licking the piece when no water is available!)
Using
a refractometer to test RI :
The most commonly used type of refractometer can measure the
RI of gems whose values fall between 1.30 and 1.80. This does
not cover the full range of gems, whose values range between
1.20 and 2.60.
When
no reading can be obtained (almost always because the RI of
the gem is above 1.80, the reading is said to be "OTL",
or over the limits), which, in itself, is often useful information
for identification purposes.
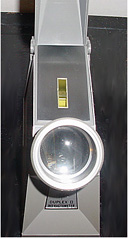
Standard
Refractometer
This
is a delicate and expensive piece of equipment which has numerous
limitations, and is difficult to learn to use properly, yet
it remains the single most useful tool for the gemologist doing
gem identifications. Most models, like the one seen above, require
a separate light source. The most important part of the device
is a leaded glass "hemicylinder", seen as the slightly
yellowish rectangle above, upon which the gem whose RI is to
be read, is placed. A drop of very high RI "contact"
fluid (methylene iodide saturated with dissolved sulfur, plus
18% tetraiodoethylene), is placed on the hemicylinder to assure
that there is no air between it and the gem.
A
system of internal mirrors reflects light which has been bent
to a specific degree by the gem, so that it falls, as a shadow,
upon a scale visible to the observer. All of this is based on
firmly established principles of mathematics and optics that
needn't concern us here.
RI
Readings :
- Can be
taken on transparent, translucent or opaque gems.
- Are best
obtained from a flat polished surface such as:
- Those
on faceted gems
- This
includes some which are set in jewelry as long as the
mounting doesn't prevent contact
- Gem
rough with at least one polished face
- Flat
polished areas of carvings or ornamental items.
Can be
estimated within a range for polished curved surfaces like those
on cabochons or carvings.
Are most
accurate when a monochromatic (one wavelength only) source of
light is used.
Are taken
with the gem in eight different postions.
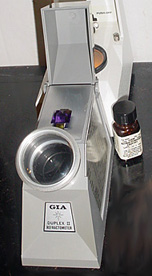
A
refractometer that is ready to take a reading
In the picture
above, a light source is in position, the recently cleaned gem
is table down on the hemicyclinder with a drop of contact fluid
in place. The picture below shows what a such a reading might
look like. The reading, shown is between 1.56 and 1.57. With
practice it is possible to be quite accurate at estimating that
third decimal place.
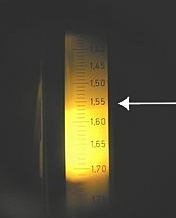
Refractometer
reading
Due to the
differential refraction of the various colors within white light,
readings obtained with white light (as seen above) are somewhat
fuzzy. Using a single wavelength source (usually yellow), produces
much sharper shadows. An SR gem will give the same RI reading
in all eight positions as it has no birefringence, but a DR gem
will give different RIs in different directions. By subtracting
the lowest from the highest, the gem's BR can be calculated.
Fluorescence :
You may recall
from the topic of selective absorption, that gems may absorb parts
of the visible light spectrum and convert them to heat. It is
also the case that gems (due to the specifics of their chemical
makeup or crystal lattices) may absorb other types of electromagnetic
radiation (UV, Xrays) and convert them to visible light. This
phenomenon is known as photoluminesence.
UV, or ultraviolet
radiation is that part of the electromagnetic spectrum that has
wavelengths just shorter than those of visible light. We divide
the UV spectrum into two parts: longwave starting at 365 nm (LW),
and shortwave starting at 254 nm (SW). Remembering that wavelength
and energy content have an inverse relationship, this tells us
that SW is the more energetic type. Although there are several
expressions of the photoluminescence phenomenon that can be tested
for in big gem labs, the type which is most useful to the average
gemologist is fluorescence testing.
Fluorescence:
When a gem absorbs either SW or LW UV, or both, and immediately
emits visible light, the phenomenon is called fluorescence. In
order to test for fluorescence it is necessary to have a controlled
source of SW and/or LW and a darkened viewing chamber. (It is
also prudent to have UV protective eyewear as exposure to these
rays can be damaging.) The specifics of the color and intensity
of fluorescence can sometimes be a useful diagnostic test in identifying
gems.
A typical
UV test lamp, as seen below, usually consists of a light source
which produces the UV with a pair of filters covering it. On
one side a filter blocks SW and permits LW to pass, and on the
other end LW is blocked passing the SW. In the model below,
a simple metal slider mechanism is moved from one side to the
other to block out the undesired wavelengths. The test would
be performed inside a viewing chamber that blocks out all visible
light. The gem to be tested must be very clean as skin oil and
dust particles often fluoresce brightly.
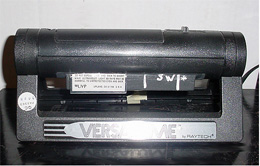
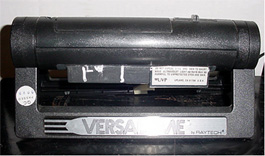
Fluoresence
tester : set up to test with SW, set up to test with LW
Fluoresence
can be absent, in which case we say the gem is inert, or present
in weak, moderate or strong form. The light emitted by fluorescence
can be the same color or a different one from the color of the
gem itself, and a gem can have the same or different reactions
to LW and SW.
-
Natural,
white to light yellow diamonds often fluoresce blue about
30% of them do so. This can be a benefit or a liability in
terms of value. In some cases the fluoresence (which happens
to a slight degree even in daylight (why?) can make a yellowish
stone look "whiter". In other cases, the effect, if strong
enough, can produce a kind of fuzzy or greasy look in the
diamond and degrade its appearance. Most often, however, it
has no noticeable effect at all. (There have been incidents
where customers have been surprised or even angry at their
jewelers when they've worn a diamond necklace or bracelet
into, say a nightclub or other situation where "blacklights"
we used, and found that some or all of their diamonds "lit
up".) Fluoresence, then, is one of the aspects important to
consider when replacing a lost diamond in a cluster setting,
and trying to match color.
-
Natural
emerald is usually inert, whereas some of the most common
types of synthetically produced emeralds fluoresce red.
-
Natural
Burmese and some Vietnamese rubies fluoresce red (even in
sunlight). They do so because of the same chromium oxide that
gives them their color. Rubies from other locales (Sri Lanka,
Africa, most areas of Thailand) also are colored by chromium
oxide, but they generally also have some iron oxide in their
chemical makeup which "dampens" or prevents the fluorescence.
The outcome of this is that a good quality ruby from a Burmese
source has a special kind of glowing red color seen in no
other gem, and it is the basis for their higher value compared
to other rubies. Synthetic rubies often show this effect even
more strongly than Burmese stones.
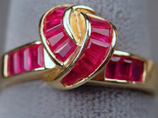
Burmese ruby ring in visible light
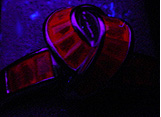
Fluorescing
red under UV (no visible light is present)
Fluorescence
testing is usually, at best, supplemental, it is rarely defintive.
There is one notable case, however, where the test can provide
proof of identity: Benitoite. There is no other blue gemstone
which shares its distinctive reaction of being inert to LW,
and fluorescing a strong, chalky blue with SW.
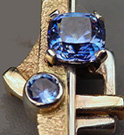
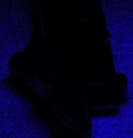
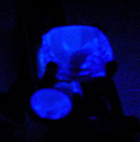
Benitoite under: visible light, LW UV, SW UV
Testing
the Absorption Spectrum of a Gem :
A "spectroscope"
contains a prism (or a diffraction grating) which serves to disperse
incoming light. This light, which has been reflected or transmitted
from the gem being tested, enters the spectroscope, and is dispersed
to display as a rainbow spectrum through the eyepiece. If significant
selective absorption has taken place, then certain portions of
that spectrum will be "missing" or reduced. Black or
darkened lines or bands indicate which wavelengths have been absorbed
by the gem and to what degree. The lines can be very distinct
and quite sharp indicating that the gem has absorbed very strongly
in a small region of the spectrum, or broad and indistinct indicating
a more general absorption over a wider band of wavelengths.
Hand held
models are relatively inexpensive, but difficult to learn to
use effectively, and to provide adequate lighting for. Desk
models are very much more expensive (the one from GIA, pictured
below, is $5000) but easier to use, and more accurate. The spectroscope
is used with a handbook of printed gem spectra for comparison.
Big labs have high tech versions, using specialized lighting
(or other energy sources, like infrared or Xray), special cooling
(liquid nitrogen), etc.
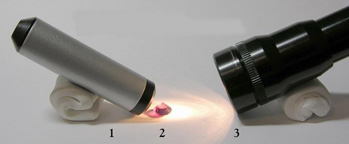
Procedure
for using a handheld spectroscope
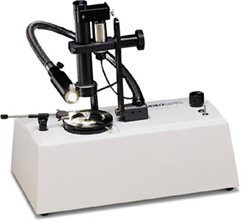
GIA
"Prism 1000"model spectroscope
By comparing
the two spectra below, it is easy to see how distinctive an
absorption spectrum can be. In such cases the spectrum may be
diagnostic of species, coloring agent or even location of origin.
Less than 25% of gem species, however, show clear, unambiguous,
absorption spectra like these. The percentage of gemologists
who can accurately use a pocket spectroscope is probably even
smaller than that! (And I cannot be counted among them.) Usually,
therefore, spectroscopic examination is a supplemental, rather
than a diagnostic, test.

Zircon
Spectrum

Almandite
Garnet Spectrum