PHYSICAL
PROPERITES OF GEMS
Properties
of Gemstones :
There
are two sets of characteristics possessed by every gemstone, and
by which they are studied, identified and evaluated.
1. Physical
properties and 2. Optical properties.
In this lesson
we'll be concerned with physical properties : those which do not
depend on the gem's interaction with light to be expressed or
measured.
All properties
of gems (whether physical or optical) derive from the underlying
three dimensional structure and chemical composition of the gem.
Or to put it another way, the chemical elements that make up the
gem, and how the atoms of those elements are put together to create
its inner structure, determine all those properties that we can
see, feel, and measure.
Amorphous
vs Crystalline :
The most basic discrimination that can be made, based on internal
structure, is that between gems which are amorphous, and those
which are crystalline.
Crystalline
gems have a specific chemical formula, and a well defined, highly
predictable internal structure, known as a crystal lattice. Amorphous
gem species also have a specific chemical formula, but their constituent
atoms are not arranged in such regular and predictable patterns
as those of crystalline materials.
Amorphous
Gems :
"Amorphous"
literally means "without form", but, of course, these
materials have a form it's just not highly regular and predictable,
nor is it expressed outwardly by the formation of crystals. Some
examples of amorphous gems are: the natural glasses, amber, jet,
opal, and "metamict" minerals.
Natural
Glasses :
The atoms making up a glass (either natural or man-made) have
been cooled from the molten state so quickly that they fail to
assume a regular crystalline pattern. A volcanic glass, like obsidian,
then, might be formed if a volcano released lava into the air
or water such that it was very rapidly cooled this very same lava
could, upon slower cooling, form a crystalline material (like
basalt, for example).
Obsidian
ranges in color from light yellow through brown to black and can
be transparent, translucent, or opaque. Those of our ancestors,
who lived in areas of volcanic activity, made ready use of these
natural glasses.
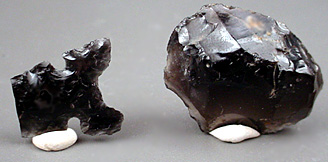
Obsidian
Artifacts
In some cases, due to the presence of other minerals
with different crystallization temperatures, when the molten material
cools, crystal inclusions may be formed. These can give the obsidian
an interesting pattern, or affect the structure in such as way
as to cause an optical phenomenon, like iridescence. Although
most obsidian is drab, single-color translucent material, two
interesting and more showy forms of this volcanic glass can be
seen below :
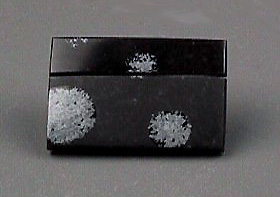
"Snowflake"
obsidian
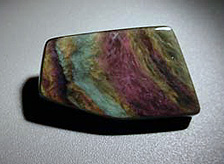
"velvet"
obsidian
Tektites :
Another
group of natural glasses, known collectively as "tektites",
are not found associated with volcanic eruptions, but rather in
places which are believed to have been sites of meteoric impact.
The heat and compression of the impacts are thought to have melted
silica sand, and the molten bits which were flung into the air rapidly
cooled into their glassy state.
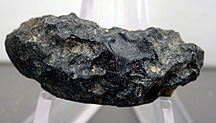
Tektite
from China
Although, like most obsidian, the various types of tektites are
dull colors of green and brown, they are still much sought after
by gem and mineral collectors. They have a following as well among
those who ascribe mystical properties to these gems, perhaps because
of their association with celestial events.
The
most commonly seen tektite is a green, near transparent type found
in the Moldau River Valley in Eastern Europe, known as Moldavite.
An intriguing light yellow form of natural glass has been found
in several areas within the Libyan Desert, and, to date, has not
been associated with a meteor impact, so its origin remains uncertain.
Moldavite
Cabochon
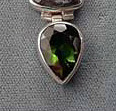
Faceted
Moldavite in Jewelry
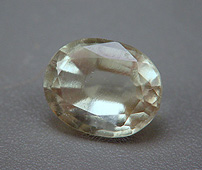
Libyan
Desert Glass
Amorphous Organics :
A
number of organic gem materials have an amorphous structure. Species
like amber and jet which are composed of organic molecules, (those
of evergreen tree resin, and the wood of certain hardwood trees,
respectively) which have been altered into a near "plastic"
polymeric state by geologic forces and time, are examples.
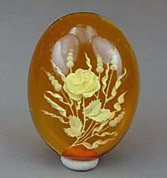
Reverse-carved
Amber Cabochon
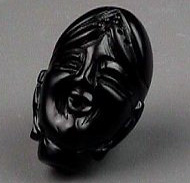
Jet
Carving
Opal
:
Although
opal is one of the most diverse gem species, with a large number
of named varieties, all share a common structural feature. An electron-microscopic
view of opal shows that it is made of row upon row of stacked silica
spheres, the exact arrangement, pattern, and size of which determine
the body color, transparency and degree of color play of the opal.
Opal
forms as a colloidial solution of tiny silica particles in water,
and as the water is lost the material solidifies to a microscopically
porous, amorphous state.
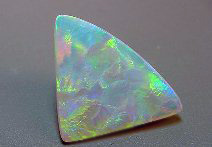
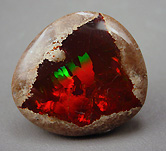
Opals from Australia and Ethiopia
Metamict
Minerals :
Most
zircon specimens that you will see, are crystalline gems, but a
few pieces (generally a dull olive green color) have lost some,
or all, of their crystalline structure, and have become disorganized
internally to a glassy state. This transformation is due to the
effects of radiation, and such a material is said to be "metamict".
The
radiation source is usually from impurities within the zircon
itself, but can be from surrounding rocks. This phenomenon can
occur naturally in several minerals, but zircon, and perhaps ekanite,
are the only ones of gem significance. These glass-like zircons
(sometimes called "low" zircons) do not have the same
super-bright luster and brilliance of the crystalline type, and
are mainly sought as curiosities by collectors.
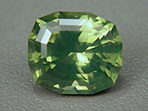
Metamict
zircon
Crystalline Gems :
The
highly regular, and sometimes startlingly angular shape of some
well formed crystals can seem eerily out of place in the world of
Nature, with its more familiar curving and flowing lines. It's no
wonder, then, that a rich history of mystical and mythological lore
pre-dates, and coexists with, today's chemical and physical understanding
of crystal structure.
Imagine
the reaction of our ancestors, so used to the shapes and forms of
flowing water, curling fire, gnarled tree branches, curving shell,
roseate flowers and sinuous leaves, when they saw something that
looks like the images below, perhaps in a mass of rock on a hillside,
or upon cracking open an ordinary looking boulder.
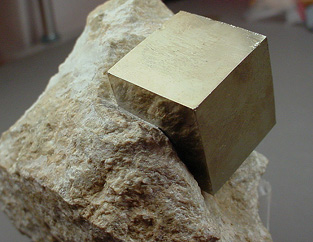
Natural
Pyrite Cubic Crystal in host rock
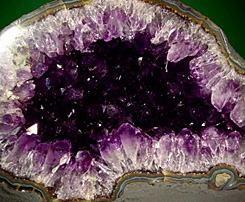
Amethyst
Crystals inside a Geode
The internal regularity that the outer features of such structures
implies, was as evident to our ancestors as it is to us, but it
was not until the beginnings of modern physics and chemistry (in
the 17th - 18th centuries) that some of the underlying causes came
to be understood. Full revelation of the most intimate details of
crystal formation and properties awaits future generations, but
major gains were made in the early 1950's with the advent of a technique
called Xray diffraction.
Although
definitely more"hi-tech", the principle behind this technique
is essentially the same as used in an ordinary medical Xray machine.
To illustrate: a beam of Xrays travels through your arm, let's say,
to Xray sensitive film below. The dense tissue (bone) absorbs more
of the Xrays than does the soft tissue, so the film is exposed differently,
and a high contrast picture is made.
In
the case of mineral crystals, the dense areas (where atoms are closer
together) absorb more Xrays than the less dense ones (where atoms
are further apart), and a high contrast picture is produced. For
those who have been trained in reading such photos, inner details
of crystal structure can be deduced by interpreting the patterns.
Single
Crystal vs Aggregate Gems :
Within the realm of crystalline gem materials, the major distinction
to be made is that between those which are composed of macroscopically
visible, single crystal units, and those which occur as a mass of
interlocking or intermeshed microscopic or submicroscopic crystals.
The
pyrite and amethysts, pictured above, are examples of single crystal
gems. If, however, you can imagine shrinking the amethyst quartz
crystals down to very, very tiny proportions and pushing them together
in random orientations such that you'd need ultra-strong magnification
to resolve them, you'd have an idea of the internal organization
of an aggregate gem, like chalcedony (an aggregate form of quartz).
Both amethyst and chalcedony are the same species: namely quartz,
so their crystals (whatever their size) are of the trigonal system,
and their chemical formula is SiO2, but the difference in the
crystal sizes and arrangement creates some notably different physical
and optical properties in the two varieties. For example, amethyst,
and other single crystal quartzes, are commonly transparent and
one color, while chalcedonies, agates, and other aggregate quartzes
are translucent to opaque and often have complex color patterns.
Although single crystal and aggregate types of quartz are equally
hard, the aggregates are notably tougher. Recall this pair of
photos from Lesson 2 which also serve as good examples here :
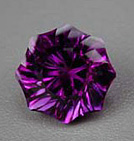
Single
crystal (amethyst)
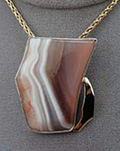
Aggregate
(agate)
Single
Crystal Gems :
Single
crystals can be large truck size or even bigger. I recall with
pleasure an undergraduate college geology field trip made to Crystal
Cave in Put-in-Bay, South Bass Island, Ohio. The cave, which was
actually an enormous underground geode, had walls and a ceiling
composed of huge celestite (or celestine) crystals.
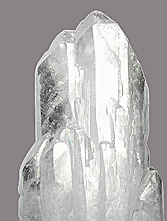
Celestite
crystal cluster from Ohio, near Crystal Cave
Single
crystal gems grow in clusters or individually, and they can be
formed within, or attached to, another mineral, or loose, as so-called
"floater" crystals. Single crystals can be quite small,
but they will still qualify as single crystals (not aggregates),
as long as they are large enough to be visible as separate entities
without high magnification. "Drusy" gems consist of
such small, to tiny, single crystals, which have grown upon a
matrix.
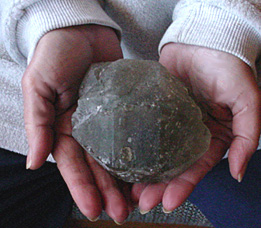
Single
Hanksite crystal
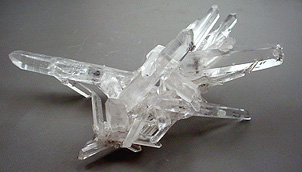
Quartz
"floater" Crystal Cluster
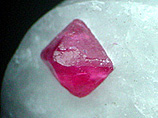
Spinel
Crystal in Calcite Marble Matrix
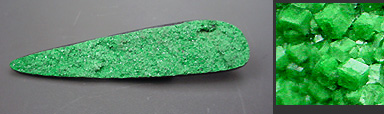
Tiny single crystals of uvarovite garnet on a matrix (drusy) with
inset showing them at 20x magnification
Aggregate
Gems: Micro- vs Crypto-crystalline :
Microcrystalline
aggregates :
Aggregates with crystals that can be resolved with a light microscope
are called microcrystalline. The standard way to view the crystals
is with a very thin slice of the gem, and about 100 - 200x magnification.
The most commonly known gem material that falls in this category
is jade.
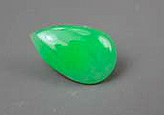
Jadeite
Jade
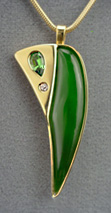
Nephrite
Jade
Cryptocrystalline
aggregates :
Aggregate quartz gems such as: agate, chalcedony, and jasper are
generally referred to as cryptocrystalline (crypto, meaning "hidden").
This is because the minute crystals cannot be resolved with a
standard light microscope, but are revealed only with an electron
microscope, or by using specialized polarizing lighting, and very
high magnifcation.
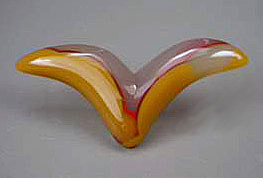
Agate
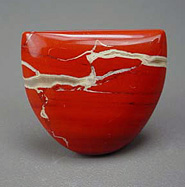
Jasper
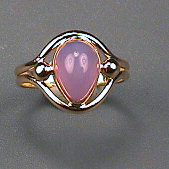
Chalcedony
Gem
Rocks :
For the sake of completeness, it should be mentioned
that although the vast majority of amorphous and crystalline gemstones
are composed of a single mineral species (other than their minor
inclusions), a few gem materials are classed as rocks. A rock
is a variable mixture of two or more minerals. Perhaps the most
familiar and valuable of the gem rocks is lapis lazuli, a mixture
of the minerals lazurite, sodalite, Hauyne, calcite and pyrite.
Other gem rocks include unakite (pink feldspar, green epidote,
and quartz) and Chinese writing stone (white feldspar crystals
in shist).
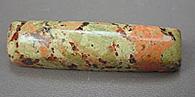
Unakite
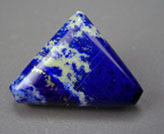
lapis
lazuli
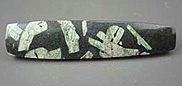
Chinese
writing stone
Crystallograpy
:
It is beyond the scope of this introductory course to delve
deeply into the complex and rigorous field of scientific crystallography,
however; it will be necessary for us to have a passing acquaintance
with a few basics. This is because the majority of gems are crystalline,
and the specific nature of their crystalline structure has bearing
on both their outward form, and their physical and optical properties.
The
Crystal Systems :
Scientific analysis has determined that there are seven basic plans
upon which all mineral crystals are built they are known as the
"crystal systems". Each of the systems has a unique architecture,
based on the lengths, and angles of intersection, of planes through
the crystal called "axes", about which there are degrees
of symmetry.
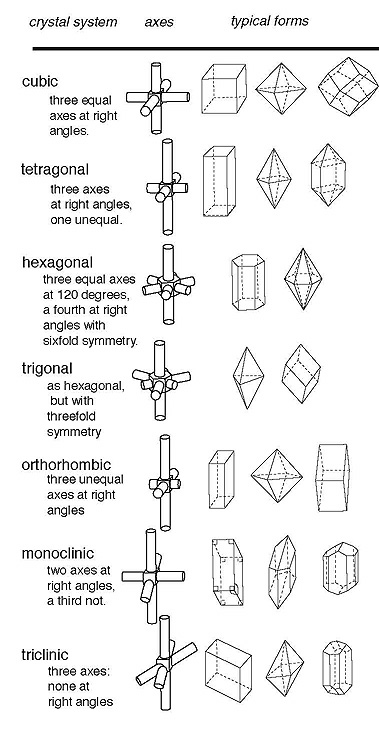
Crytstal
systems figure
Unit
Cells :
The
innermost structure of each crystal is based upon atomic-scale building
blocks that exhibit the symmetries shown in the "axes"
column in the diagram above. These tiny building blocks are called
"unit cells". The shape of a unit cell is different in
each of the crystal systems: a cube in the cubic system, a "brick"
for the tetragonal system, etc. These tiny structures assemble themselves
as the crystal grows, and build the crystal up to its finished size
and shape. It might seem, from the diagram above, that there are
only a few outward forms (or "habits") possible, given
the seven types of unit cells available but in the real world, we
find that mineral crystals come in nearly an infinite set of shapes
and sizes. How can this be?.
Because it is easiest to visualize, I'll use the cubic (also known
as isometric) system to illustrate. The unit cell in this system
is a cube: picture a baby's building block set, or (if you live
here in Nevada), dice.
Is it possible to build a big cube out of little cubes?... Sure,
just stack them up 5 x 5 x 5 or in any other equal dimenisons, and
your many little cubes become one big one. Such is the mechanism
by which the impressive pyrite cube seen earlier in the lesson was
built from the little, cube shaped, unit cells of the mineral pyrite.
It shouldn't surprise you, then, to learn that diamond (which is
also a member of the cubic crystal system) is sometimes found in
natural cubes.
Natural
diamond crystals
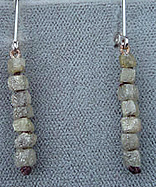
"cube" crystal habit, natural cubic diamond crystals drilled
as beads for earrings
But
using those same blocks or dice, can you build a pyramid?... You
bet! Start with a square base, and decrease each square layer
uniformly until to get to the top single cube. (5 x 5, then 4
x 4, then 3 x 3, etc). Look at the second of the "typical
forms" for the cubic system shown in the diagram above. Can
you see it as two pyramids attached to each other, base to base?
That shape is called an octahedron (meaning eight sides) and it's
a common form seen in the crystals of gems of the cubic system.
(Why are the faces of the octahedra so smooth? because the cubic
unit cells are really, really tiny, and there are enormous numbers
of them.
Fluorite
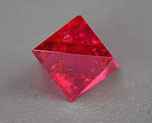
Spinel
Octahedra
Characteristic
crystal forms such as those above, that are easily recognized, and
are typical of a particular mineral, are known as its crystal "habits",
but no gem species is limited to these ideal shapes. You can also
see, I'm sure, that it's quite possible to build a random looking
structure out of your blocks or dice, one that has no readily categorizable
outer shape. This frequently seen habit in crystalline gems is referred
to simply as "massive".
Now,
recognizing that each of the seven crystal systems has a different
unit cell, and that these unit cells can be put together in many,
many ways, is there any wonder that the diversity of crystal forms
in Nature is staggering, and such a challenge, and delight to mineral
specimen collectors?
A
few of the crystal habits, due to their similarity to common objects,
are especially recognizable and have acquired special names, as
demonstrated by the specimens below :
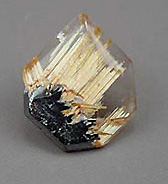
Acicular
(needle-like): golden rutile crystals in quartz'
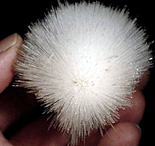
"puffball-like"mesolite
specimen of radiating acicular needles
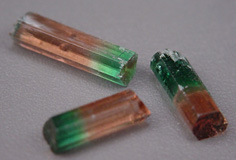
Prismatic
(pencil-like) Tourmaline Crystals
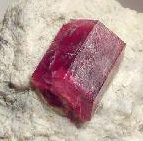
Red
Beryl Crystal in Matrix
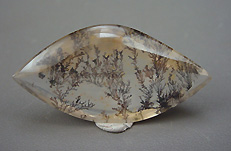
Quartz
with Black Manganese Dioxide Crystal Inclusions
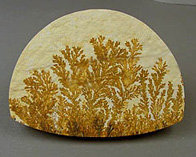
Sandstone Matrix with Iron Oxide Dendritic Crystals on surface
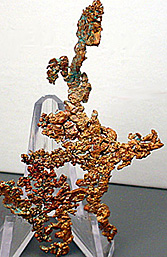
Dendritic
Native Copper Crytals
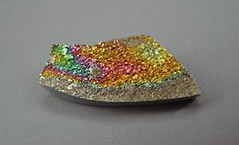
Rainbow
Pyrite Crystals on Matrix
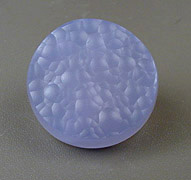
Botryoidal
(seen in aggregate gems only, like a bunch of grapes or bubbles)
: Blue Chalcedony
Crystal Growth :
Factors
affecting crystal appearance :
Although
the crystal system and unit cell which are characterisitic of a
particular gem species set certain parameters in regards to their
formation, there are also a mulitude of environmental factors that
will determine precisely what size, shape, color, and clarity a
particular crystal will have. (We'll be taking a look at how different
species of gems are formed in Lesson 10, "Gem Formation",
but regardless of the specifics, all gem formation processes are
affected by the same factors listed below).
Temperature/Pressure :
The
effect of rapid versus slow cooling of a melted material has already
been alluded to, but there is more to the story. The same molten
mass of atoms, or the same solution, or vapor of materials can crystallize
differently, depending on the temperature and pressure at the time,
and in the place, where crystallization occurs. This is because
there can be more than one stable crystal lattice composed of the
same atoms. The various stable configurations that a particular
gem species can crystallize in, are referred to as its "polymorphs".
Polymorphs :
When two materials have the same chemical formula but have crystallized
differently (due to each being subjected to different temperature/pressure
conditions at formation), they are called polymorphs. The most famous
examples are diamond and graphite. Both have the same chemical formula
(just C, pure carbon), but the "lead" in your pencil and
the diamond on your finger, obviously exhibit quite different properties.
Graphite crystals are formed of sheets of tightly bonded carbons
atoms in layers which are very loosely bound to each other, allowing
lots of slipping and sliding. Diamond crystals have each carbon
atom bonded tightly to four others surrounding it in all directions,
so the whole structure is very strong and durable.
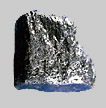
Single
Crystal Graphite and Diamond (uncut dodecahedral (12 sided)
crystal)
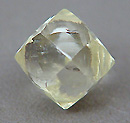
Polymorphs
of Carbon
Another
interesting gem example is the case of Al2SiO5 which can crystallize
in the orthorhombic system as Andalusite, or in the triclinic
system as kyanite.
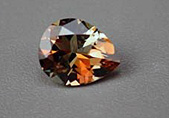
Andalusite
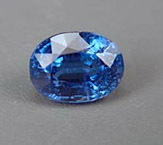
Kyanite
-
Space
available : crystals often form in cavities, cracks,
bubbles, and other cramped places, the size and shape of which
will limit growth possibilities. Some directions of potential
growth might be unavailable or limited, while others afford
plenty of "growing room". It can also occur that two or more
crystals which start growing in a space independently, can
contact and/or interpenetrate each other resulting in "twinning".
-
Chemical
elements present : Each species requires a particular
set and proportion of chemical elements for its basic makeup,
and cannot grow without them. Non-required elements, though,
which incorporate into the growing crystal in trace amounts
can have dramatic effects on the appearance (usually color)
of the gem. For example, a very small amount of the element
chromium, when present along with the necessary aluminum and
oxygen, turns, what would otherwise have been colorless corundum,
into red ruby. In addition, fluctuations in the amount or
type of growth materials present can lead to color zoning,
as well as to the creation of crystal "phantoms" and
"negative" crystals.
-
Other
minerals present : Minerals do not usually form crystals
in complete isolation. As a particular crystal is forming,
other minerals, also in the process of crystallization, can
be captured by it (to show up as inclusions) or capture it.
Exactly how this plays out will depend on the relative crystallization
temperatures and pressures required by the materials in the
group.
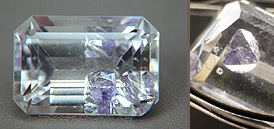
Quartz
from Madagascar with fluorite crystal inclusions, inset picture
at 10x
Special
Growth Phenomena :
Twinning
:
When growing crystals of the same mineral share one or more faces,
the result is a crystal "twin". Depending on the nature
of the twinning, which can be on either a visible, or a microscopic
scale, the shape of the crystal might be dramatically affected,
or the material's properties could be noticeably altered. Sometimes,
evidence of twinning can be seen in a crystal or cut gem due to
unusual color or inclusion patterns.
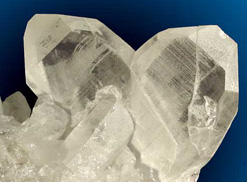
Twinned
Quartz Crystals in "rabbit ear" form
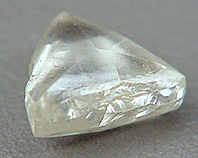
Twinned
Octahedral Diamond Crystals which form a characteristic flattened
triangular "maacle"
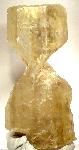
Rare
"hour glass" Twinned Gypsum Cyrstal from Australia
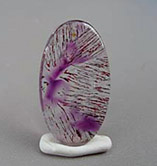
lepidocrosite
platelets of the cabochon
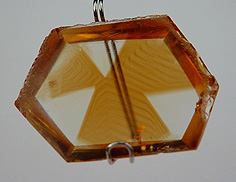
Alternating
color sectors of the Crystal slice
Phantoms and Negative Crystals :
Due
to changes in environmental conditions, starts and stops of crystal
growth occur. When other minerals, which are favored in the new
conditions, start to grow, they sometimes crystallize on the "old"
faces of the temporarily inert material. When conditions change,
and the host once again starts its growth, evidence of the pauses
may now be visibly captured as outlines of the temporary stopping
points, called "phantoms".
Likewise,
certain conditions may completely block the growth of an interior
portion of a crystal leaving a void which is bounded by the sides
of the crystal around it at first glance this "negative"
crystal looks like a solid crystal inclusion, but it is indeed empty.
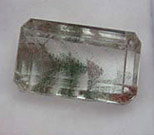
Edenite Phantoms in Quartz
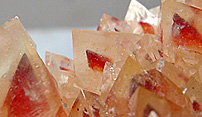
Hematite
Phantoms in Calcite
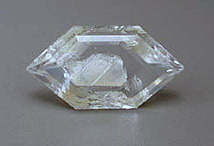
Negative
Crystal in Quartz
Seudomorphs
:
The term "pseudomorph" literally means false
form. A pseudomorph is, in a way, the opposite of a polymorph.
Whereas polymorphs are different crystal forms of the same chemical
compound, a pseudomorph shows a crystal form which is not one
recognized for its species. To put it another way, it's the case
of one mineral taking on the outward form of another while keeping
its chemistry unchanged. Let's take the example of Goethite which
is an iron oxide mineral that crystallizes in the orthorhombic
system. A glance back at the diagram for the crystal systems shows
us that orthorhombic gems do not form in perfect cubes. Pyrite,
however is an iron sulfide mineral (in the cubic system) that
frequently forms crystals shaped like perfect cubes.
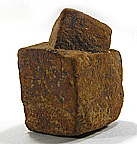
Goethite ps. after Pyrite
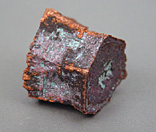
Copper
ps. after Aragonite
In
the first picture above you see what appears to be a twinned pyrite
crystal, but chemically and physically it tests not as pyrite, but
as Goethite, the second picture shows what appears to be a hexagonal
crystal, but it is made entirely of the cubic system mineral, copper.
Pseudomorphs occur when environmental conditions occur that cause
the replacement of one chemical compound with another without altering
the pre-existing three dimensional structure. Mineralogically, the
item is named as an "X" pseudomorph (ps.) after "Y".
(Goethite pseudomorph after pyrite, for example. ) Likewise those
"petrified" fossils spoken of in Lesson 1 are more technically
known as "chalcedony pseudomorphs after bone", or "opal
pseudomorphs after wood".
Chemical Groups of Gems :
In
addition to categorizing gems by their three dimensional structures,
we can also view them as belonging to various chemical groups. Due
to their related chemistries, some quite different looking gems
share some of their basic properties, while other gems which look
rather similar, differ markedly, due to their unlike chemistries.
Even if you haven't yet taken a college chemistry course, you are
undoubtedly familiar with the basic idea that the material world
around us is made of up of units (atoms) of unique substances called
elements. Examples of elements would be carbon and aluminum. These
elements are held together in crystal lattices, molecules and amorphous
materials, by attractive forces called chemical bonds.
In the world of minerals, certain groupings occur quite commonly.
For example, oxygen frequently occurs bonded to atoms of a metal
(like iron or aluminum). We call such compounds oxides, and oxide
gems have some characteristics in common. There are dozens of chemical
groups which could be listed if all gem species were taken into
account, but in this course, you will be required to recognize,
and recall, only five major groups, the: silicates, oxides, carbonates,
phosphates and native elements.
Accounting for nearly 60% of gem species, silicates are the most
important group, closely followed in prominence by the oxides. These
two groups have in common, that their member species tend to be
relatively hard and stable, while the carbonates and phosphates
are generally softer, and susceptible to attack by acids.
Recognizing which chemical group a species belongs to is simple,
as long as you know what to look for.
- Silicates
: Regardless of what other
atoms are present (usually one or more metals), gems of this
category will have in their chemical formulas some number of
Si and O (silicon and oxygen) atoms listed together as a group
. For example, as in the polymorphic species from above: Al2SiO5
here we see the group of one silicon atom and five oxygen atoms,
which identify the polymorphs Andalusite/kyanite as silicate
gems. The numbers of Si and O will vary, depending on the species,
but will always appear as a unified group.
-
In
general they tend to be hard, transparent to translucent, and
of medium density. In this very large class are all the beryls
(aquamarine, emerald, etc.), all the quartzes (amethyst, rose
quartz, agate, etc.), all the feldspars (sunstone, moonstone,
etc.), all the garnets (pyrope, Spessartite, demantoid, etc.)
topaz, tourmaline, zircon, and many other lesser known species.
Here's the first one for you : looking up emerald on page 152 you
see : Be3Al2(SiO3)6
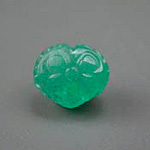
Emerald
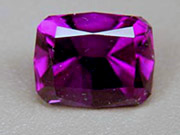
Amethyst
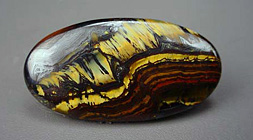
Tigers
Eye
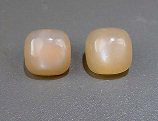
Moonstone
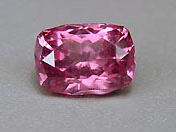
Rhodolite
Garnet
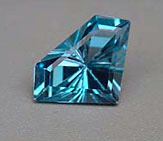
Blue
Topaz
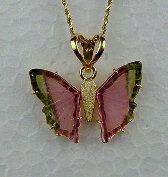
Bi-colored
Tourmaline
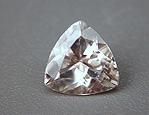
White
Zircon
-
Oxides
: This group will have one or more oxygen atoms (not
grouped with silicon, phosphorus or carbon) in their formulas.
Many oxides are important ores of metals or valuable gemstones
and tend to be quite hard and rather dense. Amongst the members
of this group are corundum Al2O3 (ruby
and sapphire), spinel, hematite and chrysoberyl.
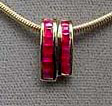
Ruby
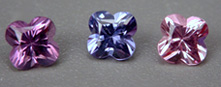
Spinel
Rhodocrosite
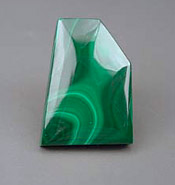
Malachite
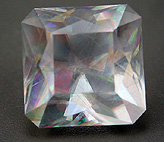
Calcite
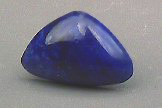
Azurite
-
Phosphates
: A PO4 group is the identifying chemical
landmark for gems of this class. Many of these gems have very
complex formulas, but do not be intimidated, you can still
see the phosphate group in there! A highly variable group,
in general they are soft, fragile, and brightly colored. Turquoise,
and apatite are notable phosphate gems.
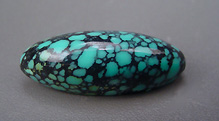
Turquoise
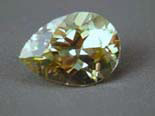
Apatite
-
Native
Elements : This is the easiest group of all to recognize,
as it consists of one and only one element. All the precious
jewelry metals such as gold, silver and platinum belong to
this group, as does diamond.
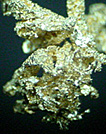
Native gold from Nevada
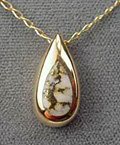
Gold
in Quartz necklace
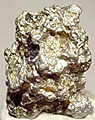
Platinum
Nugget
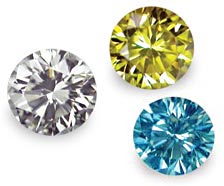
Diamonds
Two
interesting native element examples, not used as gems, but often
sought by collectors are sulfur and mercury. Pure sulfur occurs
in bright yellow crystals which would tempt the faceter if they
were not so heat sensitive that just holding them in the hand
causes them to crack, and rare native mercury has the distinction
of being the only metal found in a liquid form at normal ambient
temperatures.
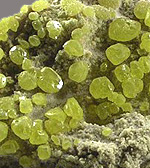
Crystals
of pure Sulfur from Mexico : beautiful to look at but too fragile
to touch
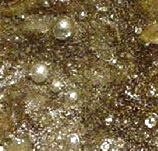
Droplets
of liquid native Mercury in matrix rock from California
Major
Physical Properties :
Although
there are a dozen or more physical properties which can be measured,
in this course we will concentrate on just a few. In particular,
our focus will be on those which are either visible directly, or
measurable with minimal equipment, and those which are most important
as indicators of a gem's identity, and/or its suitability for particular
uses :
Cleavage :
In the three dimensional structure of certain crystals, atoms are
bound more tightly to each other in some directions and more loosely
in others. As a consequence, when strong forces are applied, relatively
clean breaks may occur in these "weakest link" directions.
These breaks, which can sometimes be so smooth as to appear to have
been polished, are called cleavages. The number of directions in
which a particular material cleaves, the ease with which that happens,
and the "perfection" of the breaks are used to quantify
this characteristic.
Since cleavage, or lack of it, is a species trait, it also serves
as a good gem identification criterion. In the examples below, the
number and completeness of cleavage of three species are shown.
Species with easy or perfect cleavage, particularly when such
is the case in multiple directions, are poor risks for most jewelry
applications. Not all gems show cleavage however, for example
tourmalines, sapphires, and garnets do not.
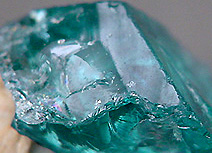
Apatite
: Two imperfect (note that cleaved surfaces are somewhat rounded
and irregular)
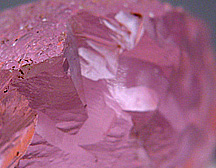
Spodumene
: Two perfect (note extremely flat, smooth breaks)
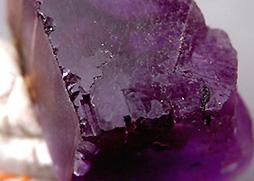
Fluorite
: Four, Perfect
Miners
have long used the cleavage properties of gems in trimming the stones
they find. "Cobbing" is the act of smacking a piece of
rough sharply and precisely with a hammer to break off any unstable
(already partially cleaved), or included areas. Knowledge of the
cleavage planes in the material being mined is essential to efficient
use of this technique.
The
use of cleavage is perhaps most well known in diamond cutting. We've
all seen photos or videos of that tense moment when the diamond
cutter inserts the wedge at a particular spot on the diamond and
strikes it with a mallet. If all goes well, the stone splits precisely
where the cutter wanted, and expected, it to. It is said that the
expert that first cleaved the (up to that time) largest rough diamond
ever found (The Cullinan) had studied it for months to determine
its cleavage planes, and upon striking the first blow fainted dead
away from anxiety. All was well, however.
Fracture
:
Whereas cleavages occur only in some gems, and within those, only
in certain directions, fractures can, and do, occur in all gems,
and in any direction. A fracture is a break which is not along a
cleavage plane. With sufficient force, any gem will fracture, although
some do so more readily than others. The edges of fractures are
not smooth like those of cleavages, but they do tend to have one
of several basic appearances.
Playing
on the resemblances of certain fracture types to well known surfaces
and objects, terms like conchoidal (shell-like), splintery, uneven,
step-like, and granular are used. Like cleavage, this is a species
specific characteristic which has value in the identification
of gems.
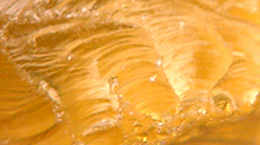
Citrine
Quartz: Conchoidal
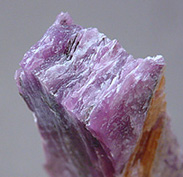
Charoite
: Splintery
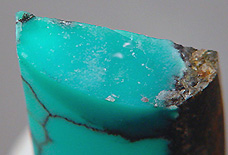
Turquoise
: Granular
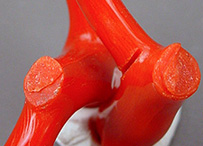
Coral
: Uneven
Conchoidal
fracture is the most common, and is found in corundum, beryls, all
the quartzes, opals, and both natural and man-made glasses. Turquoise
and coral are commonly simulated by glass.
Durability Factors :
The
concept of gem durability was introduced and described as being
made up of hardness, toughness and stability. Let's now look at
each of these factors in more detail.
Hardness :
The
tendency to resist scratching in a gem is known as its hardness.
Of the three factors comprising durability, it is the most familiar.
Even those folks with just a passing interest in gems know that
they can be ranked on a scale of hardness. Hardness is primarily
the result of the strength of the chemical bonds between the gem's
constituent atoms (how tightly they are bound to one another).
The hardness of a gem affects its wearability, luster, and resistance
to cutting and polishing. All other factors being equal, harder
gems are more useable in jewelry, develop a brighter surface luster,
and take more time and effort to cut and polish. They will retain
their polish longer than softer gems, given equal wear and tear.
The familiar 1-10 Mohs' Scale of hardness, is not an absolute measure,
but rather a relative one a kind of "pecking order". Gems
ranked at a higher number on the scale can scratch those ranked
lower, and will in turn, be scratched by those whose number is higher
than theirs.
Frederich Mohs, a 19th century German mineralogist was the originator,
and we still use his scale, with the minerals which he designated
as reference points today. For example, (softest) talc = 1, quartz
= 7 and diamond = 10 (hardest).
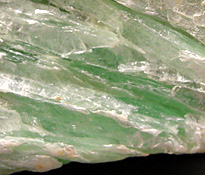
Talc,
the softest on the Mohs' scale
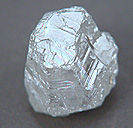
Diamond, the hardest
In
mineralogy, one of the key tests commonly used for purposes of identification
is a "scratch" test, which is done with a set of implements
known as hardness points. These, usually steel, "pencils"
are tipped with various minerals (or metals) of known hardness.
By drawing them across the surface of an unknown mineral sequentially,
the tester can determine the sample's approximate hardness. In gemology,
such tests are rarely used as they are destructive in nature. Exceptions
might be in testing the bottom of a carving, or a piece of gem rough,
or a bit of material which has broken off. Another drawback of the
standard hardness points is that they are not precise, but limited
to giving a "ballpark" estimate.
In
a laboratory setting, exquisitely precise measurements can be made
with sclerometers. These devices use diamond-tipped, hydraulically
operated probes, and can give an absolute reading on the force necessary
to penetrate the surface of a material.
Not
many hikers, nature lovers, or rockhounds carry hardness points
with around with them on their treks, but the use of just a few
ordinary materials can allow such individuals to do pretty good
hardness tests in the field.
The
Practical or Field Mohs' Scale :
1-2 : easily scratched by fingernail
3-4 : scratched by copper coin
5-6 : easily, and not so easily, scratched with pocket knife
7 : scratches window glass/scratched by steel file
8-10 : scratches window glass, but not scratched by steel file
Hardness
can be directional. This is actually quite understandable,
as it depends on chemical bonds which can differ in strength, and
in distance from each other, depending on which axis of the crystal
we are observing. Generally such differences are relatively small
and of litttle consequence, but there are two notable cases where
they are dramatic and important. 1) Kyanite is notoriously difficult
to cut because of its extreme directional hardness differences.
2) Diamond cutting would scarcely be possible unless the cutters
could use the directional hardness of that gem to their advantage.
Soft
Gems :
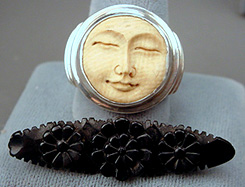
Ivory
and Jet : 2.5
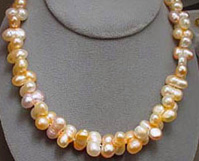
Pearl
: 3
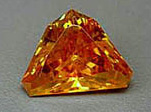
Sphalerite : 3.5
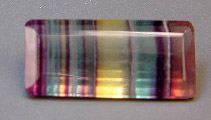
Fluorite
: 4
Gems
of intermediate hardness :
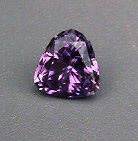
Scapolite
: 6
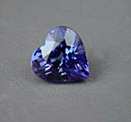
Tanzanite
: 6.5
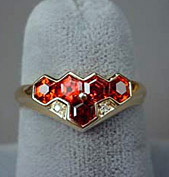
Garnet
: 7 - 7.5 depending on species
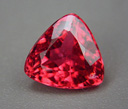
Tourmaline
: 7.5
Hard
Gems :
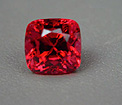
Spinel
: 8
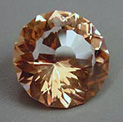
Topaz
: 6.5
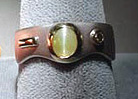
Chrysoberyl
: 8.5
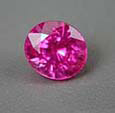
Sapphire
: 9
Toughness
:
The tendency to resist breaking and chipping is known as a gem's
toughness. This property is controlled primarily by two factors:
the readiness of a material to cleave in single crystal gems, and
the presence or absense of certain structural characteristics in
aggregate and/or amorphous gems which promote strength and cohesion.
All
other factors being equal, the harder the gem, the tougher it will
be, but all other factors are not always equal. Take the case of
topaz, for example. At hardness 8 it seems to be a pretty rugged
gem, but if we consider its strong tendency to cleave in one direction,
in reality, it is rather fragile.
Likewise,
diamond, the "star" of the hardness game, is only ranked
as "good" when it comes to toughness because of its
cleavage and fracture potential. Diamonds are usually cut with
a flat culet facet at the tip of their pavilion, rather than coming
to a sharp point as do colored stones. This is due to the likelihood
of a fracture (or cleavage) in the fragile culet zone. When purchasing
a diamond it is a good idea to check the girdle under magnification
to make sure that it is not excessively thin, as this is another
site of special vulnerability. Likewise, the corners and points
of cuts like baguettes, trillions and marquis are vulnerable,
and should be protected by the mounting when used in jewelry.
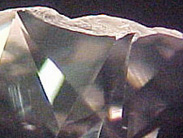
Fracture
on the Girdle of a Diamond
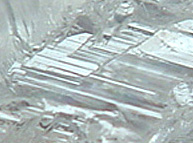
Cleavages
on Diamond with classic "staircase" pattern
On
the other hand, nephrite jade with its hardness of 6.5 might seem
to be delicate, but due to the felted, fibrous nature of its aggregate
crystals, it is literally the toughest gem on Earth! So it is with
pearls, which with their extremely low hardness, would barely be
wearable at all, except for their moderately good toughness. It
results from the layered, overlapping nature of the aragonite mineral
plates of which the pearls are made, and the proteinaceous "mortar"
that holds these brick-like layers together.
Toughness affects both wearability and resistance to polishing.
Jade gems thousands of years old are as beautiful today as when
they were first made. A well polished jade is a sign of a dedicated
and skillful lapidary, as its structural characteristics make it
susceptible to "undercutting" and an "orange peel"
surface effect if not handled expertly and with patience.
There
is no numeric scale on which toughness is measured, rather, relative
terms such as: exceptional, excellent, good, fair and poor are used.
Fragile
Gems :
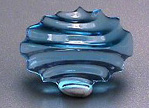
Topaz
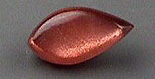
Sunstone
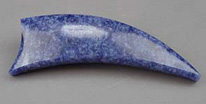
Sodalite
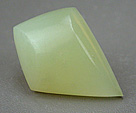
Serpentine
Gems
of intermediate toughness :
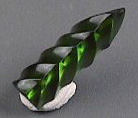
Tourmaline
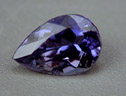
Iolite
: fair
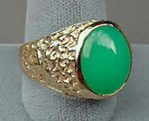
Chrysoprase
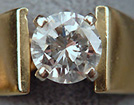
Diamond
: good
Tough
Gems :
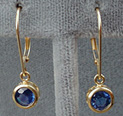
Sapphire
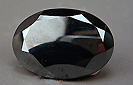
Hematite
: excellent
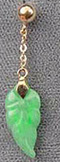
Jadeite
Jade
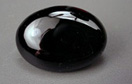
Nephrite
Jade : exceptional
Stability
:
Stability
in a gem is a measure of its ability to resist changes due to exposure
to light, heat and/or chemicals. Not only does stability affect
wearability, but it also dictates appropriate ways of fashioning,
cleaning and storing the gems. Most gems are stable, but a few (even
some quite popular ones) are unstable, and must be handled accordingly.
The
Effects of Heat :
Dehydration :
Heat is a factor that can create problems with certain gems. In
some cases, the mineral comprising the gem is "hydrated",
that is, it contains water molecules which adhere chemically with
varying degrees of tenacity. When the water is rather loosely attached,
hot dry air can lead to loss of some of the water, and changes in
the color, or transparency of the gem. Even more seriously, its
loss can cause a network of cracks to form in the gem, in a process
called "crazing". Opal is the most well known gem for
which this is an issue.
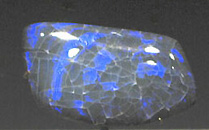
Badly
crazed Opal
It
is sometimes suggested that opal gems and jewelry items be stored
in water or oil when not being worn this is NOT good advice. Water
will not hurt the opal, but it will not help it either. The type
of "chemically linked" water that is lost when crazing
occurs cannot be replaced by soaking, nor can this procedure be
used as a preventative.
It
is the structural details of the particular type of opal, including
the percentage of water in it, that determine the likelihood of
crazing. Reputable opal dealers "proof" their material
before it is sold, by subjecting it to hot dry conditions for months.
Generally, those pieces that survive such treatment will be stable
under normal wearing conditions.
(Leaving an opal on a car dashboard for hours in the August sun,
or forgetting that your opal ring is in your pants pocket, and then
putting the pants in the dryer for an hour on high, would NOT be
examples of normal wearing conditions! Soaking in oil is an especially
bad idea as opal is a porous gem and the oil seeps inside and then
discolors over time, degrading the gem's beauty.)
Thermal
Expansion :
Another problem that heat creates for some gems is caused by their
inherent capacity for "thermal expansion". This is a yet
another physical characteristic by which gems differ. Diamond is
notably stable to temperature changes (with slow and even rates
of thermal expansion), so much so, that jewelers can pour molten
metal into molds containing wax models with the diamonds already
in place, to cast pre-set jewelry pieces.
Other
gems, such as apatite, expand so rapidly with sharp rise in temperature,
that their crystal structure is damaged, and they crack or even
shatter. Heat sensitivity of that degree makes it very important
for lapidaries cutting such gems, and jewelers working on mountings
containing them, to keep the gem cool during these processes.
The
Effect of Inclusions :
Although a gem might be quite temperature stable itself, inclusions
of other minerals within it, could have different degrees of thermal
expansion from their host. This situation becomes quite important
in the heat treatment processes used to enhance gems. Internal inclusions
can literally explode or, less dramatically, expand, and in doing
so, create internal "stress cracks" in the gem being treated.
(For this reason, it is standard practice among Tanzanite heat treaters
to heat only cut stones which have had virtually all the inclusions
removed, and to avoid heating rough material.)
To
an extent, heat treaters can ameliorate such effects by very,
very, slowly raising and lowering temperatures. Tanzanite heaters
might take 12 to 24 hours to incrementally reach the desired temperature,
hold the gems there for several hours, and then take another 12
to 24 hours to gradually cool them down. At the highest temperature
levels, though, such as those required to heat treat corundum,
or those used for "color diffusion" processes, nothing
can prevent heat damage. This is good news in a sense, though,
because such internal and external cues to the heating, help the
jeweler or gemologist spot the gem as one which has been subjected
to extreme temperatures.
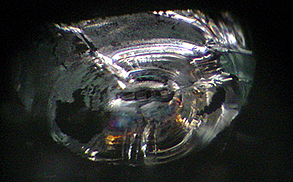
In
the center of this picture of the interior of a gem under high
magnification, you see an included, heat shattered crystal, broken
into four pieces, and a series of stress fractures surrounding
it positive evidence of high heat treatment in this gem
There
are cases where thermal expansion characteristics of gems are
used to deliberately induce cracks or stress
fractures. Pieces of amber which have been heated, and then quickly
cooled, develop disk-like stress fractures called "sun spangles"
which some consider to be attractive.
"Sun
Spangles", stress fractures in heated Amber
A
very old method of dyeing gems, which is still occasionally used
today, is called "quench crackling" single crystal gems,
like quartz, for example, which would ordinarily not absorb dye
are heated and plunged in cold water to fill them with cracks
that, then, can take up the dye, giving apparent color to the
whole piece.
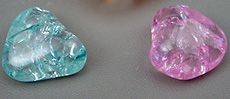
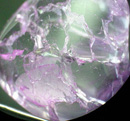
Quench-crackled quartz pebble dyed pink, the closeup shows clearly
that the pink dye is confined just to the cracks
Other
Environmental Factors :
Light
:
Some gems can fade or change color when exposed to light. An extreme
example of this phenomenon is seen in the rare mineral pyrargyrite
which must be kept constantly under opaque covers or else light
exposure quickly renders its originally red color completely black.
In the case of gem minerals, there are only a few to be concerned
about. Kunzite (pink spodumene) can lighten in color with long term
exposure to bright light, and is sometimes suggested as an "evening
only" gem. Certain brown topazes, notably those from Mexico,
can lighten dramatically, even becoming colorless with continuous
light exposure.
Chemicals
:
Exposure to various chemicals can ruin the polish of, and/or discolor
certain gems. Two important cases would be carbonate gems, like
rhodocrosite, which degrade due to a chemical reaction when exposed
to acids, and amber which can be dissolved by acetone. It is doubtful
that a drop of lemonade, or vinagrette salad dressing, or a bit
of spilled nail polish remover would harm such stones, but acid
vapors found in the polluted air of many cities can take their toll
over time, as can some intense solvents, such as paint strippers,
which might be used in the home or workplace. A dip in certain jewelers'
solutions, like the hot "pickle" used to remove oxidation
from metals, would be devastating to rhodocrosite, while a few hours
spent soaking in "AttackTM" (a solvent used to remove
glues used in jewelry making) would ruin an amber gem.
Most
gems in the unstable category, however; are sensitive more in virtue
of their porosity, than because of their chemical makeup. Pearls
and turquoise are two gems well known for their propensity to absorb
cosmetics, perfumes, body oils, sweat, etc., and to dull and discolor
as a result. Often fine turquoise gems are given a final polish
with a layer of colorless paraffin wax to help seal and protect
them from such degradation.
Lightly
wiping chemically sensitive gems with a damp cloth after each wearing
will help to keep them in good shape. Any gem which is suspected,
or known, to be chemical or heat sensitive should be protected from
steam or solvent cleaning methods. Such considerations also become
a factor in gemological testing in that, turquoise, for example,
cannot be placed in the chemicals that would be used to determine
specific gravity, or those used in relative refractive index testing.
Unstable
Gems :
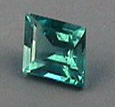
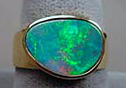
Apatite
and Opal : heat sensitive
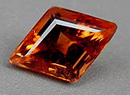
Mexican
Brown Topaz : fades in light
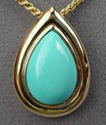
Turquoise
: porous and likely to discolor with exposure to various materials
Specific
Gravity :
Specific
gravity, also known as relative density, differs widely among gemstones,
and is one of their most important physical characteristics from
the viewpoint of gem identification. Specific gravity (SG) is the
ratio of the weight of one unit volume of the gem to the weight
of the same unit of water. For example, to say sapphire (corundum)
has SG = 4.0, means precisely that a cubic inch of sapphire weighs
four times as much as a cubic inch of water. In natural gems, SG
values range from just over 1 (1.08 for amber) to just short of
7 (6.95 for cassiterite).
Light
Gems SG < 3.O :
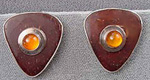
Amber
: 1.08
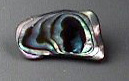
Shell
: 1.30
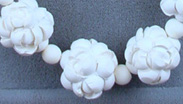
Meerschaum
: 1.50
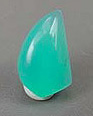
Opal
: 2.10
Medium
Density Gems : SG : 3 - 4 :
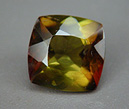
Andalusite
: 3.16
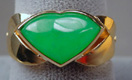
Jadeite
: 3.33
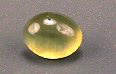
Chrysoberyl
: 3.71
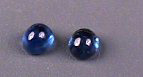
Sapphire
: 4.00
Heavy
Gems SG>4 :
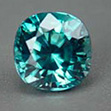
Zircon
: 4.69
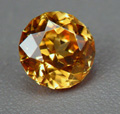
Scheelite
: 6.10
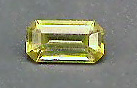
Anglesite
: 6.35
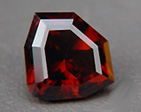
Cassiterite
: 6.95
A
curious student might ask at this point : "Why do specific
gravities differ so much?" The answer, satisfyingly, goes back
to the basic premise of this lesson (that all physical properties
of gems are the result of their chemical and structural makeup).
The
various elements of which gems are made have atoms of different
weights. Atoms of gaseous elements like hydrogen and oxygen are
light, while metallic elements like aluminum and iron have heavy
atoms. Chemists use "atomic weights" to describe elements
rounding them off, here are some examples: hydrogen = 1, carbon
= 12, oxygen = 16, aluminum = 27, silicon = 28, calcium = 40, iron
= 56, zinc = 65, and lead = 207.
It's
quite logical, then, that a cubic inch block of lead is going to
weigh much more than a cubic inch block of aluminum. Extending that
idea to gems, we can see that if a gem is made of relatively heavy
elements it will have a greater SG than if it is made of lighter
ones.
There
is a second factor to consider, however; which is the structure
: How are those atoms put together? Are they tightly packed or loosely
arrayed? The examples below will help to illustrate the interplay
of chemical make-up and crystal structure in determining specific
gravity.
1. First, let's look at the case where structure is held constant
but atomic makeup is different. Here, we'll compare two minerals
that have the same crystal structure, in this case they both are
of the orthorhombic system, and, they have identical chemical formulas
except for substitution of one element for another.
Calcite : CaCO3 V.S. Smithsonite : ZnCO3
Both consist of five atoms per unit: either a Ca or a Zn plus one
carbon and three oxygens. Both are put together with the "atomic
packing" characteristic of the orthorhombic system of crystals.
Their SGs differ though, with calcite = 2.71 and Smithsonite = 4.35.
Looking at the list above and seeing that calcium's atomic weight
is 40 and that of zinc is 65 gives us our answer.
2.
Now to examine the effect of structure, by holding the chemical
makeup constant... Remembering the concept of "polymorphs"
from the first part of this lesson, we'll compare calcite and aragonite.
Both have the same chemical formula, CaCO3 :
Calcite : orthorhombic crystal system -vs- aragonite
: trigonal crystal system
Both are made up of the same elements in the same proportions, but
those building blocks are put together differently so their SGs
differ, with calcite = 2.71 and aragonite = 2.94
Measuring
Specific Gravity :
Although
SG measurements can be made on either rough or cut gems, the gems
must be unmounted, and composed of a single material. You cannot
do a SG measurement on a gem that is set in a piece of jewelry,
or on an assembled stone, like a doublet. Porous gems cannot be
measured with at least two of the techniques, as the liquid they
absorb affects the SG measurement, and, in some cases, can harm
the stone. Detailed reference books meant for mineralogists or gemologists
will list SGs for gems as a range, rather than a single number,
due to the fact that individual specimens will differ slightly based
on the number and type of their inclusions. (Your texts, meant for
non-professional use, however, use a single number average of the
SG range for the gem species). There are several ways in which SG
is measured, and they differ in precision as well as suitability
to different gems and circumstances.
Hefting
:
The crudest technique, but one that can be rather useful in some
situations, is simple hefting. By lifting the gem and gently throwing
it up in the air and catching it, a general feel for its density
can be gained. This technique is often all that is needed to discriminate
plastic and some glass imitations from the much denser gems they
mimic. Conversely, jewelers who are intimately familiar with the
heft of a 6.5 mm diamond (which will weigh almost exactly one carat)
may be able to quickly pick out a 6.5 mm imposter because so many
diamond simulants have SGs substantially higher or lower than diamond.
Heavy
Liquids :
For most of us, though, in most circumstances, hefting would not
supply enough information. One popular method is based on the principle
of bouyancy: "an object will sink in a fluid of lesser SG,
remain suspended in one of equal SG, and float in one of higher
SG." This technique uses a set of "heavy" liquids
with known SGs. By immersing the unknown gem material in the liquids,
and observing its behavior, its approximate SG can be deduced.
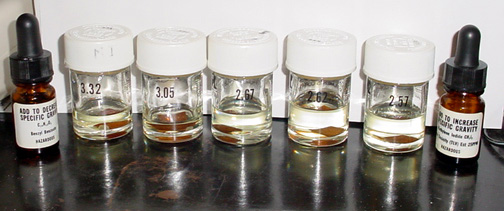
Heavy
Liquids Testing Set, SGs of liquids are printed on bottles, dropper
bottles are for calibration
To
give a simple example, consider an unknown gem that floats quickly
in the 3.05 bottle, sinks rapidly in th 2.57 bottle, and floats
and sinks very slowly in the 2.67 and 2.62 bottles, respectively.
That would tell you that the SG was between 2.67 and 2.62 and would
allow you to rule out a great many minerals and focus any further
tests on a smaller group of "possibles". Corundum (SG
= 4.0) would behave quite differently from these observations, and
could be excluded, while quartz, whose SG is 2.65 would behave precisely
as described, and could not, therefore, be excluded.
Hydrostatic
Weighing :
By far the most precise technique for SG determination involves
use of a specially modified weighing balance that allows a gem sample
to be weighed in air (Wa), and also weighed in water (Ww). Using
Archimedes Principle : "a body immersed in water weighs less
by the volume of water displaced", and a simple calculation,
SG can be determined with substantial accuracy.
SG calculation : Weight of gem in air divided by
the difference between the weight in air and the weight in water,
or : SG = Wa/ Wa-Ww
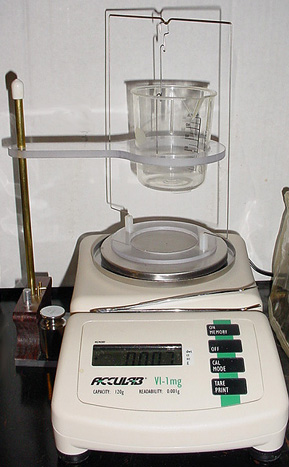
Hydrostatic
weighing set-up consisting of an electronic balance with a special
hanging basket apparatus in which the gem can be suspended in
water without putting weight on the scale
Again,
an example. We have an unknown gem whose weight in air is 5.10
ct and whose weight in water = 3.20 ct. The difference in the
air and water weights is 1.90 ct. Using the formula: SG = 5.10
ct/1.90 ct = 2.68. Looking in the tables at the back of the Hall
book we quickly find several gem possibilities close to that SG:
quartz (2.65), coral (2.68), aquamarine (2.69), and scapolite
(2.70). More importantly, than what it might be, a SG of 2.65
rules out a large number of possibilities that it cannot be. The
gemologist, like other scientists, progresses most often by weeding
out wrong hypotheses (as opposed to proving right ones!).
Scenario :
We have obtained an unknown transparent green gem from a jeweler,
the label has fallen off the box, and he/she would like us to
tell them what it is. Since the gem was going to be used for jewelry,
we can rule out the obscure and very soft collector gems, and
limit our scope to relatively common jewelry gems that come in
vivid, transparent green. This leaves emerald, chrome diopside,
Tsavorite garnet and tourmaline as the prime suspects. We are
just getting our gemology laboratory off the ground, so all we
have is some reference books, a hydrostatic weighing set up, and
a set of heavy liquids. First, we'll do our SG test hydrostatically,
then with the heavy liquids.
Answer :
We look up the SG ranges in our reference guides.
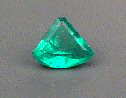
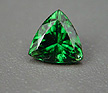
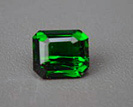
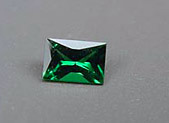
Is
it?: Emerald (SG = 2.72 +.18/-.05); Tourmaline (SG = 3.06 +.20/-.06);
Chrome Diopside (SG = 3.29 +.11/-.07) or Tsavorite Garnet (SG
= 3.61 +.12/-.04)
Hydrostatic
Test :
Step One : Weigh Gem in Air.
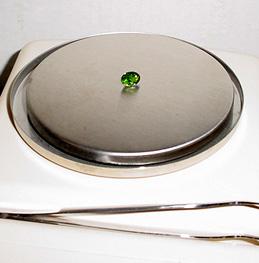
The
weight in air is: 2.420 ct.
Step
Two : Assemble hydrostatic weighing chamber and "tare"
balance.
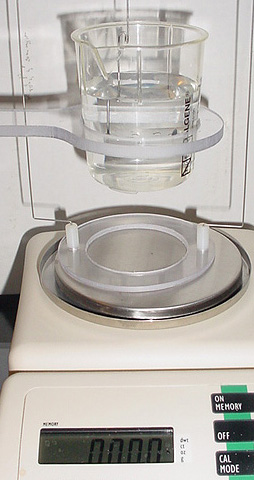
The
beaker with water is actually suspended by an arm off to the side
and does not put weight on the balance pan, the plastic ring which
holds a little metal basket for the gem, does put weight on the
balance, though. Once everything is set up, we "tare"
the balance (resetting it to zero) so that it ignores the weight
of the plastic ring and gem basket. Now we are ready to place
the gem in the basket where it will be weighed underwater.
Step
Three : Weigh Gem in water.
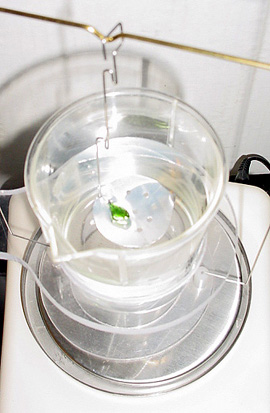
The
weight of the gem in water is: 1.615 ct. The difference between
the weight in air and weight in water is: 2.420 ct - 1.615 ct
= 0.805 ct
Step
Four : Calculate SG.
SG = Wa/ Wa-Ww
SG
= 2.420 ct /0.805 ct = 3.01
Can
we eliminate any possibilites? Check the SG range of each of the
four possibilities. (Assume we have made accurate measurements and
our arithmetic is correct).
Emerald
(SG = 2.72 +.18/-.05); Tourmaline (SG = 3.06 +.20/-.06); Chrome
Diopside (SG = 3.29 +.11/-.07) or Tsavorite Garnet (SG = 3.61 +.12/-.04)
The
only gem whose SG range does not exclude 3.01 is: Tourmaline! The
others are either too high or too low to qualify.
Testing
by Heavy Liquids : Below are the results of the same test on the
green gem, done with the set of heavy liquids :
3.32 : gem floats rapidly
3.05 : gem floats very slowly
2.67 : gem sinks
2.62 : gem sinks rapidly
2.52 : gem sinks very rapidly
Based on these results, the conclusion we must draw is that the
SG is below 3.05, and above 2.67 (but closer to 3.05). If this were
the only available testing method, we would be able to eliminate
the chrome diopside and the Tsavorite garnet, but we'd have to do
some other tests to discriminate between emerald and tourmaline.
Most
gemologists prefer to use the hydrostatic method, not only because
of its greater precision, but also because the heavy liquids smell
very bad, and have hazardous properties such that gloves and masks
must be worn when using them.
Home
made heavy liquid test for Amber :
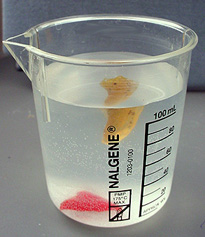
A
saturated solution of salt water with amber and plastic immersed
One
fun, and safe, heavy liquid test that can be done at home uses a
saturated saltwalter solution. (Make this by dissolving as much
salt in room temperature distilled water as it will hold). The SG
of this mini "Salt Lake" is about 1.13. Most types of
natural amber will float in it (SG = 1.08) while nearly all the
plastic materials used to make imitations of amber will sink as
their SGs are higher than 1.13. Imitation amber is rampant in the
gem marketplace (even in some of the better stores), so this is
a handy trick to know.
Miscellaneous physical properties :
There is a very long list of esoteric physical properties which
gemologists / physicists / crystallographers and others working
in research labs can study and measure in gems and minerals. To
finish up this section, though, I will mention just a few that have
occasional usefulness for the ordinary gemologist or gem/jewelry
lover, and that do not require high budget equipment.
1. Magnetism :
Very few gems show any magnetic properties. One interesting exception
is a certain type of synthetic diamond. In this case, a strong magnet
can be a definitive way to separate these stones from natural diamonds.
Natural hematite is mildly to moderately attracted to a magnet,
but an imitation version is so strongly magnetic that the difference
is obvious.
2. Thermal Reaction :
The response to high temperature in terms of appearance, and especially
odor, can be a telling one in identifying some gems. Many organic
gems such as horn, ivory, tortoise shell, and black coral smell
like burning hair when touched with a "hot point" probe.
Amber smells like turpentine, and jet like burning coal. Their common
imitations may have odors, but not the right ones.
Although, technically destructive, this test can usually be done
on a very small, inconspicuous spot. Resin, lacquer and wax coatings
on gems can likewise be detected as they melt or char under the
hot point. In this case, the reaction is best observed under magnification,
with the hot probe not touching the surface, but just barely above
it.
3. Thermal Conductivity :
Gems differ quite dramatically in this property, which is basically
a measure of the rate at which they conduct applied heat. For many
years no savvy jeweler or pawn shop owner would be caught without
a thermal conductivity tester (otherwise known as a diamond tester).
By simply touching a small metal probe to the gem, it was instantly
determined to be "diamond" or "not diamond".
Pretty useful, huh? Well, it used to be.
A few years ago, two developments occured which have all but made
these devices obsolete. 1) A new diamond simulant, called Moissanite
whose thermal conductivity is close enough to diamond to pass the
test, has come on the market, and 2) synthetic diamonds are now
becoming a common enough to be concerned about. Man-made diamonds
which have the same physical properties as the natural gems, would,
of course, pass the test as diamond.
4. Electrical Conductivity :
Very quickly upon the heels of the introduction of Moissanite, came
the marketing of a new generation of testers which use a different
tactic to separate Moissanite from diamond. Diamonds (with the vanishingly
rare exception of natural blue ones) do not conduct electricity,
but Moissanite does. So, out with the old and in with the new generation
of diamond testers.
These machines have two systems, a thermal conductivity test, to
first separate diamond and Moissanite from all other gems, then
an electrical conductivity test to do the final separation should
the thermal test indicate diamond. (Again, synthetic diamonds cannot
be separated from natural ones with any basic physical tests).
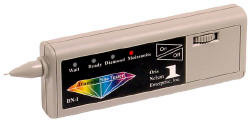
Mizar DiamonNite Dual Tester
Placing
the probe on a gem initiates a thermal conductivity test to reveal
CZ or other non-diamond simulants, then if the stone passes that,
an electrical conductivity test follows to determine if it is
diamond or Moissanite.